陶明生,任延杰,黄杰,周立波,邱玮,黄伟颖,李聪,陈荐,牛焱
(长沙理工大学 能源与动力工程学院,长沙 410114)
摘要:
为了进一步揭示增材制造对金属材料的微观组织与高温氧化性能的影响规律,本文采用光学显微镜、扫描电子显微镜、能量色散谱、电子背散射衍射和X射线衍射等方法,对比研究了轧制态与激光选区熔化(Selective Laser Melting,SLM)制备的 Inconel 625合金垂直和平行于成形方向横截面(XY和XZ面)的微观结构,并探究了两种合金在900 ℃下的高温氧化性能。研究表明,SLM制备的合金与传统轧制合金的显微组织存在明显区别:轧制合金呈等轴晶,晶粒尺寸为(15±2.5) μm,具有更多的大角度晶界和较大的位错密度;SLM制备的合金呈多晶结构,主要由胞状晶与柱状晶组成,晶粒尺寸不均匀,其中胞状晶晶粒尺寸为0.2~2 μm,位错密度较小,呈现高度织构化特征;XRD结果表明,SLM并未改变合金的物相,SLM与轧制成形 Inconel 625合金由γ-Ni相组成。SLM合金的XY面和XZ面的晶粒取向存在较大差别,其中XZ面的晶粒取向为(001)。在900 ℃下,SLM合金的氧化速率更高,这种高氧化速率导致氧化膜致密性差,在SLM合金的亚表层区域形成空洞。轧制Inconel 625合金的抗氧化性能优于SLM合金,这主要归因于轧制合金具有更多的位错与孪晶。
关键词: 激光选区熔化成形 轧制 Inconel 625合金 显微组织 高温氧化
DOI:10.11951/j.issn.1005-0299.20220049
分类号:TB31
文献标识码:A
基金项目:国家自然科学基金面上项目(52171066,52175129);湖南省教育厅重点项目(21A0204).
Microstructure and high temperature oxidation properties of Inconel 625 alloy fabricated by rolling and selective laser melting
TAO Mingsheng,REN Yanjie,HUANG Jie,ZHOU Libo,QIU Wei,HUANG Weiying,LI Cong,CHEN Jian,NIU Yan
(College of Energy and Power Engineering, Changsha University of Science & Technology, Changsha 410114, China)
Abstract:
The influence of additive manufacturing on the microstructure and high temperature oxidation properties of metal materials was investigated. As-rolled and selective laser melting (SLM) formed Inconel 625 alloy blocks were prepared. The microstructures of the cross-sections (perpendicular and parallel to the forming directions, namely XY plane and XZ plane) of alloys were comparatively analyzed by means of optical microscope (OM), scanning electron microscope (SEM), energy dispersion spectroscopy (EDS), electron backscatter diffraction (EBD), and X-ray diffraction (XRD). The high temperature oxidation properties of the two alloys at 900 ℃ were studied. Results show that the microstructure of the alloy prepared by SLM was obviously different from that of traditional rolled alloy. The rolled alloy presented a microstructure of equiaxed grains with grain size of (15±2.5) μm, and there were more high-angle grain boundaries and higher dislocation density. The alloy prepared by SLM presented a polycrystalline structure, which mainly consisted of cellular crystal and columnar dendrite; the grain size was uneven: the cellular crystal grain size was 0.2~2 μm, and the dislocation density was smaller with highly textured characteristics. XRD results show that SLM did not change the phase of the alloy, and both materials had the phase structure of γ-Ni phase. There was a great difference in grain orientation between XY plane and XZ plane of SLM formed alloy, and the grain orientation was (001) for XZ plane. At 900 ℃, the oxidation rate was higher for alloys prepared by SLM, which induced poor oxide scale compactness, as well as void formation in the subsurface region of alloys. The oxidation resistance of rolled Inconel 625 alloy was better than that of SLM formed alloy, which was mainly attributed to the fact that the rolled alloy had more dislocations and twins.
Key words: selective laser melting forming rolling Inconel 625 alloy microstructure high temperature oxidation
陶明生, 任延杰, 黄杰, 周立波, 邱玮, 黄伟颖, 李聪, 陈荐, 牛焱. 激光选区熔化与轧制Inconel 625合金的微观组织与高温氧化性能研究[J]. 材料科学与工艺, 2022, 30(4): 1-10. DOI: 10.11951/j.issn.1005-0299.20220049.

TAO Mingsheng, REN Yanjie, HUANG Jie, ZHOU Libo, QIU Wei, HUANG Weiying, LI Cong, CHEN Jian, NIU Yan. Microstructure and high temperature oxidation properties of Inconel 625 alloy fabricated by rolling and selective laser melting[J]. Materials Science and Technology, 2022, 30(4): 1-10. DOI: 10.11951/j.issn.1005-0299.20220049.

基金项目 国家自然科学基金面上项目(52171066, 52175129);湖南省教育厅重点项目(21A0204) 通信作者 任延杰,E-mail:yjren@csust.edu.cn 作者简介 陶明生(1996—),男,硕士研究生;
牛焱(1961—),女,教授,博士生导师,国家杰出青年基金获得者 文章历史 收稿日期: 2022-02-18 网络出版日期: 2022-06-22
Contents Abstract Full text Figures/Tables PDF
激光选区熔化与轧制Inconel 625合金的微观组织与高温氧化性能研究
陶明生, 任延杰


长沙理工大学 能源与动力工程学院,长沙 410114
收稿日期: 2022-02-18; 网络出版日期: 2022-06-22
基金项目: 国家自然科学基金面上项目(52171066, 52175129);湖南省教育厅重点项目(21A0204)
作者简介: 陶明生(1996—),男,硕士研究生;
牛焱(1961—),女,教授,博士生导师,国家杰出青年基金获得者.
通信作者: 任延杰,E-mail:yjren@csust.edu.cn.
摘要: 为了进一步揭示增材制造对金属材料的微观组织与高温氧化性能的影响规律,本文采用光学显微镜、扫描电子显微镜、能量色散谱、电子背散射衍射和X射线衍射等方法,对比研究了轧制态与激光选区熔化(Selective Laser Melting,SLM)制备的Inconel 625合金垂直和平行于成形方向横截面(XY和XZ面)的微观结构,并探究了两种合金在900 ℃下的高温氧化性能。研究表明,SLM制备的合金与传统轧制合金的显微组织存在明显区别:轧制合金呈等轴晶,晶粒尺寸为(15±2.5) μm,具有更多的大角度晶界和较大的位错密度;SLM制备的合金呈多晶结构,主要由胞状晶与柱状晶组成,晶粒尺寸不均匀,其中胞状晶晶粒尺寸为0.2~2 μm,位错密度较小,呈现高度织构化特征;XRD结果表明,SLM并未改变合金的物相,SLM与轧制成形Inconel 625合金由γ-Ni相组成。SLM合金的XY面和XZ面的晶粒取向存在较大差别,其中XZ面的晶粒取向为(001)。在900 ℃下,SLM合金的氧化速率更高,这种高氧化速率导致氧化膜致密性差,在SLM合金的亚表层区域形成空洞。轧制Inconel 625合金的抗氧化性能优于SLM合金,这主要归因于轧制合金具有更多的位错与孪晶。
关键词: 激光选区熔化成形 轧制 Inconel 625合金 显微组织 高温氧化
Microstructure and high temperature oxidation properties of Inconel 625 alloy fabricated by rolling and selective laser melting
TAO Mingsheng, REN Yanjie


College of Energy and Power Engineering, Changsha University of Science & Technology, Changsha 410114, China
Abstract: The influence of additive manufacturing on the microstructure and high temperature oxidation properties of metal materials was investigated. As-rolled and selective laser melting (SLM) formed Inconel 625 alloy blocks were prepared. The microstructures of the cross-sections (perpendicular and parallel to the forming directions, namely XY plane and XZ plane) of alloys were comparatively analyzed by means of optical microscope (OM), scanning electron microscope (SEM), energy dispersion spectroscopy (EDS), electron backscatter diffraction (EBD), and X-ray diffraction (XRD). The high temperature oxidation properties of the two alloys at 900 ℃ were studied. Results show that the microstructure of the alloy prepared by SLM was obviously different from that of traditional rolled alloy. The rolled alloy presented a microstructure of equiaxed grains with grain size of (15±2.5) μm, and there were more high-angle grain boundaries and higher dislocation density. The alloy prepared by SLM presented a polycrystalline structure, which mainly consisted of cellular crystal and columnar dendrite; the grain size was uneven: the cellular crystal grain size was 0.2~2 μm, and the dislocation density was smaller with highly textured characteristics. XRD results show that SLM did not change the phase of the alloy, and both materials had the phase structure of γ-Ni phase. There was a great difference in grain orientation between XY plane and XZ plane of SLM formed alloy, and the grain orientation was (001) for XZ plane. At 900 ℃, the oxidation rate was higher for alloys prepared by SLM, which induced poor oxide scale compactness, as well as void formation in the subsurface region of alloys. The oxidation resistance of rolled Inconel 625 alloy was better than that of SLM formed alloy, which was mainly attributed to the fact that the rolled alloy had more dislocations and twins.
Keywords: selective laser melting forming rolling Inconel 625 alloy microstructure high temperature oxidation
镍基高温合金以其较低的热膨胀系数、优异的加工性能和抗高温氧化性能被广泛应用于航空航天、能源动力、冶金设备等领域[1-4]。20世纪50年代美国国际镍公司为了满足高负荷下主蒸汽管道对材料的需求,以Ni-Cr合金为基础,添加Mo、Nb以及少量的Ti、Al、Mn、S等元素制成了Inconel 625合金。Inconel 625高温合金属于固溶强化变形的高温合金,因其良好的耐高温腐蚀性能[5-6],以及良好的疲劳性能和蠕变性能[7],现已被广泛应用于制造航空航天发动机零部件、石油设备、化工厂的热交换器和核工业中的化工设备[8-10]。
现代增材制造技术在生产复杂精细构件方面具有独特的优势,结合结构拓扑优化技术,能够显著减轻零部件质量[11]。作为增材制造技术的一个分支,激光选区熔化(SLM)技术因其制造过程中的高精度和低孔隙率的特点而得到了迅速的发展[12]。目前,以增材制造方式制造镍基高温合金的技术日渐成熟,学者们对增材制造镍基高温合金的微观组织展开了较多的研究。Ramenatt等[13]采用激光熔凝法(LBM)逐层制造的Inconel 625合金呈柱状晶结构,其晶格参数略大于锻造合金,而晶粒尺寸则略小于同成分的锻造合金。于雁东等[14]发现SLM过程中,扫描速度降低会使形成的Inconel 625合金的枝晶减少,胞状晶增加,晶粒尺寸降低。Li等[15]发现,SLM制备的Inconel 625合金组织主要为奥氏体,未发现析出相。熔池由细长柱状的晶体组成,且由于Nb、Mo等元素的过饱和程度较高,SLM成形的金属具有较高的的硬度(343HV)。Antonsson等[16]和DuPont等[17]提出,SLM成形镍基合金过程中,Nb在γ相中的溶解度随着冷却速率的增加而降低。
微观结构上的差异会进一步影响合金的抗氧化能力。Ramenatte等[13]发现,LBM成形的Inconel 625合金的抗氧化性受表面质量和微观结构的影响,使得抗氧化性接近或低于同成分的锻造合金。Kim等[18]发现,退火处理可以使得SLM技术制备的IN738LC合金中析出Ni3(Al, Ti)相,合金局部化学成分变化,提高了氧化层中保护性氧化铝的含量,相对于未经退火处理的增材制造合金,抗氧化性能有所改善。Sun等[19]发现,激光扫描能量密度会影响SLM Inconel 625合金的表面形态以及微观结构,从而改变合金的抗氧化性。
增材制造Inconel 625合金微观组织与传统制造同成分合金存在明显区别[5, 20-21],其高温氧化性能也有所不同。本文将采用光学显微镜(OM)、扫描电子显微镜(SEM)及电子背散射衍射(EBSD)方法研究轧制和SLM(XY和XZ面)制备Inconel 625合金的晶粒形貌、织构和位错密度等微观结构,探索选区激光技术制备合金的成形机理,并探讨微观结构对Inconel 625合金高温氧化性能的影响。
1 实验1.1 实验材料轧制Inconel 625材料是德国BGH Edelstahl Freital Gmbh公司制造的直径为40 mm的坯料,并经退火(980 ℃/50 min/淬火)处理,其化学成分见表 1。激光增材制造原料为气雾化的商用Inconel 625粉末,成分见表 1,成形效果见图 1,其中横截面(XY面)为SLM的水平扫描面,纵截面(XZ面)为SLM的垂直建造面。
表1(Table 1)
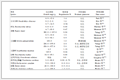
Ni Cr Mo Nb Fe Si Ti Mn C Others
轧制 63.22 21.80 8.61 3.62 1.66 0.25 0.22 0.20 0.05 0.15
SLM 63.25 22.21 8.65 2.25 1.86 0.53 0.26 0.40 0.20 0.11
表 1 两种Inconel 625合金的化学成分(质量分数/%)Table 1 Chemical composition of two Inconel 625 alloys (wt.%)
图 Figure1(Fig.Figure1)
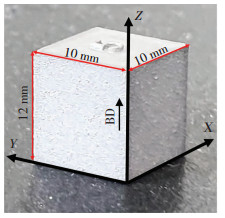
1.2 微观表征方法将轧制与SLM成形的Inconel 625合金材料切割成尺寸为10 mm×10 mm×5 mm的样品,其中SLM成形合金按照水平和垂直方向切出XY和XZ面。砂纸逐级打磨至2 000#后用1.5 μm的金刚石抛光剂抛光,用无水乙醇清洗后吹干,采用HCl(15 mL)+HNO3(5 mL)腐蚀Inconel 625样品,腐蚀时间为20 s。腐蚀后用无水乙醇清洗,并用样品夹具沿样品厚度方向夹持,使用Axiovert 200 MAT的德国蔡司金相显微镜和捷克TESCAN MIRA4 LMH电子扫描显微镜观察合金的微观组织。利用德国Bruker D8A A25 X射线衍射仪(XRD)对SLM和轧制制造合金进行物相分析。测试参数为:铜靶,电压40 kV,电流40 mA,扫描角度范围(2θ)10°~110°,步长为0.02(°)/s。使用牛津仪器NordlysMax3电子背散射衍射(EBSD) 系统测定晶粒取向、晶粒尺寸和晶界特征,所得数据利用Channel 5软件进行分析。
1.3 高温氧化实验在900 ℃下,使用SEARAM TAG1750热天平进行等温氧化实验,其灵敏度为0.1 μg,纯O2的流速为100 mL/min,炉内O2压力为1 atm。样品在20 K/min的升温速率下加热至900 ℃。每次测试结束后,炉子以5 K/min的降温速率降至室温。氧化后的合金用环氧树脂冷镶固定。打磨抛光后,利用SEM、能量色散X射线光谱仪(EDS)和XRD对氧化后合金的氧化产物层的微观结构进行表征。
2 结果与讨论2.1 金相组织图 2(a)为轧制Inconel 625合金的金相组织形貌,可以看到轧制合金的显微结构由等轴晶组成,晶粒尺寸为(15±2.5)μm,为典型的奥氏体形态,具有大量细变形孪晶。由图 2(b)可见,晶界中有少量的析出相。EDS分析表明:析出物(图 3(a))富含C(47.4at.%)、Ni(26.8at.%)、Cr(12.2at.%)、Mo(7.3at.%)和Nb(3.3at.%)。与基体(图 3(b))中的C(22.2at.%)、Ni(49.0at.%)、Cr(20.2at.%)、Mo(4.1at.%)和Nb(1.7at.%)相比,C、Mo和Nb的含量显著增加,证明析出物为碳化物。
图 Figure2(Fig.Figure2)
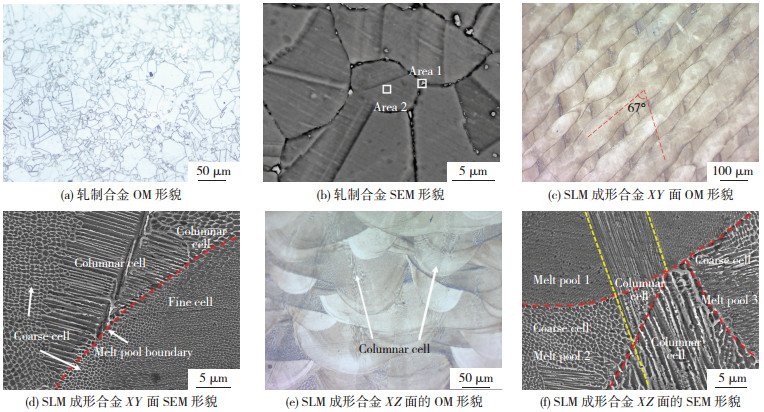
图 Figure3(Fig.Figure3)
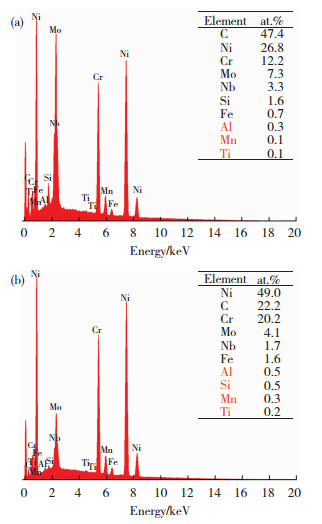
图 2(c)为激光选区熔化制备Inconel 625合金的XY平面的金相显微组织,可观察到激光束交叉扫描的痕迹特征,经测量角度约为67°。显微组织中呈现出长度不等的熔道,这种独特组织的形成原因是激光照射在粉末表面将颗粒熔化,形成大量微小的熔池,熔池相互重叠、粘结,形成熔池通道。熔池通道之间没有明显的过渡区,扫描间距约为110 μm。合金无裂纹、气孔等缺陷,层间直接形成良好的冶金结合。图 2(d)为激光选区熔化制备的XY面的表面形貌。按晶粒特征可分为3个区域:熔池内部较细小的胞状晶、熔池边界处较粗大的胞状晶和垂直于熔池边界生长的柱状晶,这与文献中的结果一致[22-23]。由于激光选区熔化成形过程中凝固速度快,使得组织和晶粒比轧制合金更加细小。在成形过程中,相邻两条熔道产生的重叠区域会经过两次熔化过程,导致晶粒再次长大,因此熔池边界处的晶粒尺寸较大。
图 2(e)为激光选区熔化制备Inconel 625合金的XZ平面的金相组织形貌。由于成形过程中激光能量的高斯分布,导致熔池呈弧形鱼鳞结构平行分布XZ面上[24-26]。形成这种结构的原因是激光束作用于粉末的区域为圆形光斑,由于大部分激光能量集中在激光束中心,而边缘的能量密度低,当输入熔池的线能量密度(激光功率/扫描速度)增大时,较高的热量输入使得底层沉积层上部再次熔化形成熔池,能量输入越高,形成熔池的深度和宽度将会越大。因此,冷却凝固后熔化道横截面呈弧形分布。在构建方向上观察到柱状晶垂直于熔池边界生长(如图 2(e)所示),这是由于加工过程中的温度梯度、凝固过程中奥氏体的择优生长方向以及晶粒的外延生长所致[15, 27]。柱状晶在垂直于具有较大温度梯度方向的熔池边界生长。图 2(f)为激光选区熔化制备的XZ平面的表面形貌。与图 2(d)类似,晶粒主要由不同尺寸的胞状晶以及柱状晶组成。在图 2(f)中发现蜂窝状晶体,尺寸在0.2 μm(熔池1)到2 μm(熔池2)变化。这是由于成形过程中熔池形成的对流导致了合金的微观结构复杂多样。
此外,图 2(d)、(f)中并未发现明显的Laves析出相,这是因为Laves相的数量、形态和大小受温度梯度(G)与固液界面迁移速度(R)之比(G/R)的影响。较高的扫描速度导致的高冷却速率和高G/R比将减少Laves相的析出,并使微观结构更加均匀[28]。
2.2 物相分析图 4为轧制和SLM(XY面和XZ面)制备的Inconel 625合金的XRD衍射谱图。轧制合金典型组织主要由面心立方结构的γ相(基体相)组成。轧制合金中由于碳化物相和金属间化合物的晶粒尺寸小、含量少,未出现第二相衍射峰[3, 15]。结合XRD与微观形貌(图 2(c)~(f)),激光选区熔化合金物相仅为奥氏体相,这是因为SLM成形过程中激光束移动很快,且熔池的凝固速率极高,液相中的溶质原子固溶于奥氏体中,形成单一的γ-Ni[29-30]。与轧制合金的晶面取向相比,SLM成形合金中的晶粒沿(111)生长趋势减弱,在(200)和(220)增强。其中,在XY方向上,晶粒沿(200)生长的趋势较XZ方向更明显;在XZ方向上,晶粒沿(220)生长的趋势更明显,这与文献[31-34]中的结果一致。
图 Figure4(Fig.Figure4)
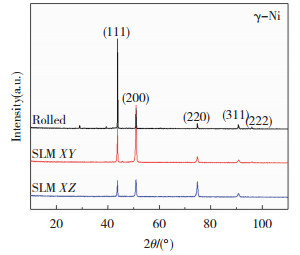
2.3 织构分析为了进一步对比分析显微组织的差异,对轧制和SLM (XY面和XZ面)制备Inconel 625合金进行EBSD分析。图 5为合金的晶粒取向分布图,其中不同颜色的区域代表晶粒的不同类型的取向。由图 5(a)可见,轧制Inconel 625合金以近等轴晶为主,晶粒较小,拥有较多的孪晶,与金相观察的结果一致(图 5(b))。SLM制备的Inconel 625合金XY面(图 5(c))的熔池内部晶粒主要呈(101)和(001)取向。SLM制备的Inconel 625合金XZ面(图 5(d))熔池内部晶粒分布较为复杂,柱状晶粒从熔池底部向顶部穿过熔池生长,表现为较强的织构特征。而成形过程中上下相邻两层的扫描方向发生了变化,使得热流/传热方向发生了偏转,改变了柱状晶生长方向,形成转向枝晶。
图 Figure5(Fig.Figure5)
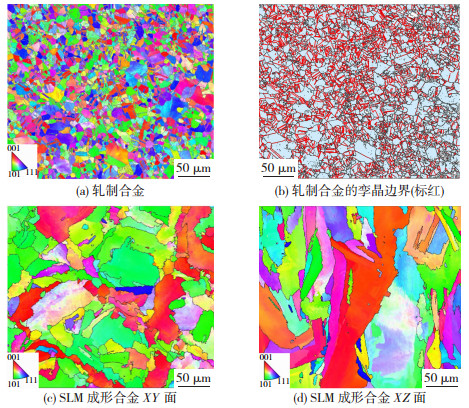
为了进一步了解SLM以及轧制的Inconel 625合金的织构分布,对图 5进行进一步分析得到反极图(图 6)。对于各向同性的材料,理论织构指数等于1×random,对应各向异性的材料,织构指数会大于1×random[35],织构强度通常为织构指数的平方根。
图 Figure6(Fig.Figure6)
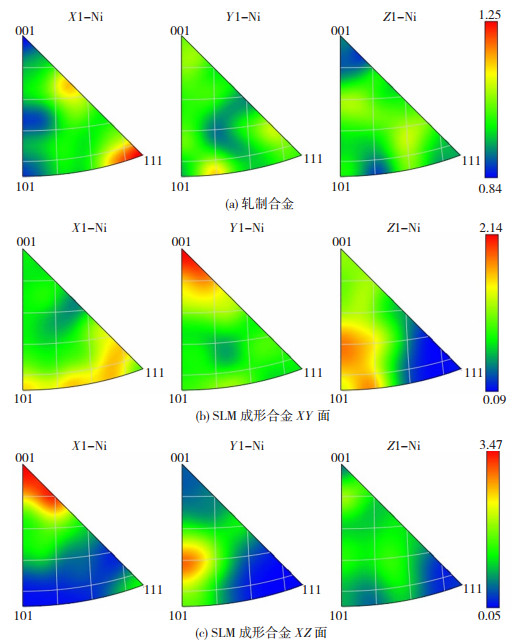
由图 6(a)可见,轧制合金在(111) 方向上具有较强的取向,织构指数为1.25× random。SLM合金由于择优取向呈现较强的(001)取向,XY面和XZ面的织构指数分别为2.14×random、3.47×random。其中,在XZ面上(001)的织构指数最大,这是由于γ-Ni属于面心立方体结构,在SLM成形过程中,晶粒沿(001)方向散热最快[36],晶体的生长方向与垂直建造方向平行,当激光束扫描粉末层时,柱状晶粒顶部重新熔化,在Z轴方向上形成从上到下的温度梯度。同时,由于熔池内的高温容易导致过热,并进一步导致在熔池中难以形成均匀形核,从而促进了柱状晶在熔池中的生长[15]。
图 7为合金的KAM图(Kernel Average Misorientation),主要用于估算合金的位错密度[37-38]。通常,位错分布在晶界附近。由图 7可见,SLM合金的位错密度远小于轧制合金,其中,XY面和XZ面上的位错密度相近。由于Inconel 625合金为低层错能合金,轧制成形后有大量的孪晶产生(图 5(b)),面心立方晶体孪生晶面为{111},母体与孪晶晶粒有 < 111>/60°的取向差关系[39],使得轧制成形合金表面晶粒取向差角约为60°,所占比例最大(如图 7(d)所示)。SLM成形后的合金,晶粒取向差(如图 7(e)、(f)所示)主要分布在20°~55°,与轧制合金相比,取向差为60°的晶界含量显著减少,孪晶数量显著降低。
图 Figure7(Fig.Figure7)

2.4 高温氧化性能图 8为两种方法制备的Inconel 625合金在1atm O2中900 ℃下氧化50 h的动力学曲线。如图 8(a)所示,在900 ℃下,氧化0~9 h内SLM合金的氧化增重小于轧制合金。随着氧化时间的延长,轧制合金的SLM样品的氧化增重超过轧制合金。两种合金的氧化过程均经历两个抛物线阶段(图 8(b)),其中轧制合金的第1氧化阶段(0~9 h) 的氧化速率kp1=5.88×10-13 g2/(cm4·s);第2氧化阶段(9~50 h)的氧化速率发生了明显下降,kp2 =2.10×10-13 g2/(cm4·s)。这可能是因为在轧制样品表面生成了一层保护性的氧化膜,降低了氧化速率。SLM合金的第1氧化阶段(0~16 h) 的氧化速率kp1=1.11×10-12 g2/(cm4·s);第2氧化阶段(16~50 h)的氧化速率降低,为kp2=3.11×10-13 g2/(cm4·s)。SLM合金的氧化第1阶段长于轧制合金,表明轧制合金形成的氧化膜更加致密。
图 Figure8(Fig.Figure8)
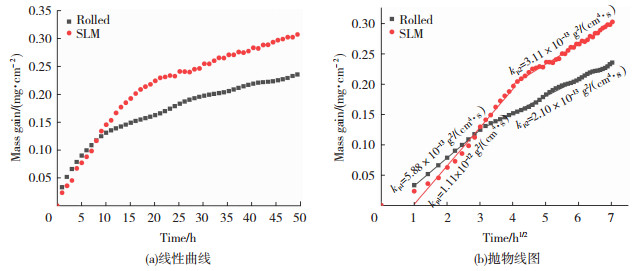
图 9为轧制和SLM Inconel 625合金在氧气中,高温氧化50 h后的截面微观形貌及EDS谱图。图 10为合金在900 ℃氧化50 h后表面的X射线衍射谱图。在900 ℃下,两种方法制备的合金外氧化膜均为单层结构,主要由氧化铬构成。轧制合金的氧化膜较为致密,厚度约3.5 μm;SLM合金的氧化膜较为疏松并发生部分剥落,厚度约为4.5 μm。在金属/氧化膜界面均观察到δ-Ni3Nb生成。此外,轧制合金发生了Al的内氧化,深度为6~7 μm。在SLM合金的氧化膜下方区域, 则发现了少量空洞存在(图 9(b)),这是由于Cr从合金向氧化膜的迁移导致了大量的空位,空位的积累可能会导致形成空洞,也被称为Kirkendall孔[13]。文献[40]的研究表明,Cr扩散产生的空位被位错消除。由于轧制合金有着较大的位错密度,故轧制合金中未观察到空洞(图 9(a))。有文献[37, 41]表明,合金中的位错也会促进Cr扩散,有利于保护性氧化铬膜的生长。轧制的Inconel 625合金的位错密度显著高于SLM成形合金,在氧化初期即可形成致密的氧化膜,因此。在900 ℃的高温环境下,轧制合金的抗氧化性能优于SLM合金。
图 Figure9(Fig.Figure9)
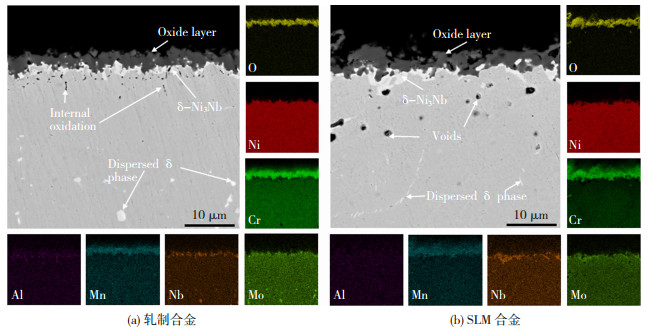
图 Figure10(Fig.Figure10)
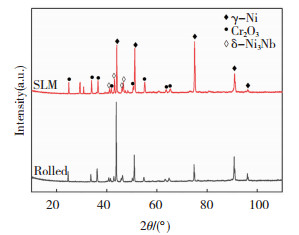
3 结论1) 轧制Inconel 625合金为典型的奥氏体形态,具有大量细变形孪晶和偏析,有碳化物第二相存在。SLM制备的Inconel 625合金熔池内部为较细小的胞状晶、熔池外为较粗大的胞状晶和垂直于熔池边界生长的柱状晶。SLM成形合金的熔池内部晶粒尺寸在0.2~2 μm范围内。
2) 轧制合金在(111)方向择优生长,SLM成形合金在XZ面晶粒织构特征最强,(001)取向最强。SLM制备Inconel 625合金位错密度小于轧制合金。轧制合金的晶界取向差主要在60°分布,而SLM合金主要分布于20°~55°范围内。
3) 在900 ℃下,轧制Inconel 625合金的抗氧化性能优于SLM合金,轧制合金较高的位错密度有利于提高Inconel 625合金的抗氧化性能。
参考文献
[1] GUO Q M, LI D F, GUO S L, et al. The effect of deformation temperature on the microstructure evolution of Inconel 625 superalloy[J]. Journal of Nuclear Materials, 2011, 414(3): 440-450. DOI:10.1016/j.jnucmat.2011.05.029
[2] GARCIA-FRESNILLO L, CHYRKIN A, B?HME C, et al. Oxidation behaviour and microstructural stability of alloy 625 during long-term exposure in steam[J]. Journal of Materials Science, 2014, 49(17): 6127-6142. DOI:10.1007/s10853-014-8344-7
[3] XU F J, LV Y H, LIU Y X, et al. Microstructural evolution and mechanical properties of Inconel 625 alloy during pulsed plasma arc deposition process[J]. Journal of Materials Science & Technology, 2013, 29(5): 480-488. DOI:10.1016/j.jmst.2013.02.010
[4] GANESH P, KAUL R, PAUL C P, et al. Fatigue and fracture toughness characteristics of laser rapid manufactured Inconel 625 structures[J]. Materials Science and Engineering: A, 2010, 527(29-30): 7490-7497. DOI:10.1016/j.msea.2010.08.034
[5] STASZWSKA K, SCENDO M. Mechanism and kinetics oxidation of Inconel 617 and 625 alloys[J]. Technical Issues, 2016(1): 82-89.
[6] HUCZKOWSKI P, LEHNERT W, ANGERMANN H H, et al. Effect of gas flow rate on oxidation behaviour of alloy 625 in wet air in the temperature range 900~1 000 ℃[J]. Materials and Corrosion, 2017, 68(2): 159-170. DOI:10.1002/maco.201608831
[7] EVANS N D, MAZIASZ P J, SHINGLEDECKER J P, et al. Microstructure evolution of alloy 625 foil and sheet during creep at 750 ℃[J]. Materials Science and Engineering A, 2008, 498(1-2): 412-420. DOI:10.1016/j.msea.2008.08.017
[8] MITTRA J, DUBEY J S, BANERJEE S. Acoustic emission technique used for detecting early stages of precipitation during aging of Inconel 625[J]. Scripta Materialia, 2003, 49(12): 1209-1214. DOI:10.1016/S1359-6462(03)00488-3
[9] 张小伟. 金属增材制造技术在航空发动机领域的应用[J]. 航空动力学报, 2016, 31(1): 10-16.
ZHANG Xiaowei. Application of metal additive manufacturing in aero-engine[J]. Journal of Aerospace Power, 2016, 31(1): 10-16. DOI:10.13224/j.cnki.jasp.2016.01.002
[10] 张永忠, 石力开, 章萍芝, 等. 激光快速成形镍基高温合金研究[J]. 航空材料学报, 2002(1): 22-25.
ZHANG Yongzhong, SHI Likai, ZHANG Pingzhi, et al. Research on laser direct deposition of nickel base superalloy[J]. Journal of Aerospace Materials, 2002(1): 22-25. DOI:10.3969/j.issn.1005-5053.2002.01.005
[11] GU D D. Laser Additive Manufacturing of high-performance materials[M]. Verlag: Springer, 2015.
[12] 张立浩, 钱波, 张朝瑞, 等. 金属增材制造技术发展趋势综述[J]. 材料科学与工艺, 2022, 30(1): 42-52.
ZHANG Lihao, QIAN Bo, ZHANG Chaorui, et al. Summary of the development trend of metal additive manufacturing technology[J]. Materials Science and Technology, 2022, 30(1): 42-52. DOI:10.11951/j.issn.1005-0299.20210111
[13] RAMENATTE N, VERNOUILLET A, MATHIEU S, et al. A comparison of the high-temperature oxidation behaviour of conventional wrought and laser beam melted Inconel 625[J]. Corrosion Science, 2020, 164: 108347. DOI:10.1016/j.corsci.2019.108347
[14] 于彦东, 李磊, 胡海亭. 选区激光熔化成形Inconel 625高温合金的工艺及性能[J]. 热加工工艺, 2020, 49(24): 21-25, 30.
YU Yandong, LI Lei, HU Haiting. Process and properties of inconel 625 super alloy prepared by selective laser melting[J]. Hot Working Technology, 2020, 49(24): 21-25, 30. DOI:10.14158/j.cnki.1001-3814.20173789
[15] LI S, WEI Q S, SHI Y S, et al. Microstructure characteristics of Inconel 625 superalloy manufactured by selective laser melting[J]. Journal of Materials Science & Technology, 2015, 31(9): 946-952. DOI:10.1016/j.jmst.2014.09.020
[16] ANTONSSON T, FREDRIKSSON H. The effect of cooling rate on the solidification of INCONEL 718[J]. Metallurgical & Materials Transactions B, 2005, 36(1): 85-96. DOI:10.1007/s11663-005-0009-0
[17] DUPONT J N, LIPPOLD J C, KISER S D. Welding metallurgy and weldability of Nickel-base alloys[M]. New Jersey: John Wiley & Sons, 2011.
[18] KIM K S, YANG S, KIM M S, et al. Effect of post heat-treatment on the microstructure and high-temperature oxidation behavior of precipitation hardened IN738LC superalloy fabricated by selective laser melting[J]. Journal of Materials Science & Technology, 2021, 76: 95-103. DOI:10.1016/j.jmst.2020.11.013
[19] SUN Y, CHEN L, LI L, et al. High-temperature oxidation behavior and mechanism of Inconel 625 super-alloy fabricated by selective laser melting[J]. Optics & Laser Technology, 2020, 132: 106509. DOI:10.1016/j.optlastec.2020.106509
[20] DE SOUSA MALAFAIA A M, DE OLIVEIRA R B, LATU-ROMAIN L, et al. Isothermal oxidation of Inconel 625 superalloy at 800 and 1 000 ℃: microstructure and oxide layer characterization[J]. Materials Characterization, 2020, 161: 110160. DOI:10.1016/j.matchar.2020.110160
[21] HUCZKOWSKI P, LEHNERT W, ANGERMANNH H, et al. Effect of gas flow rate on oxidation behaviour of alloy 625 in wet air in the temperature range 900~1 000 ℃[J]. Materials and Corrosion, 2017, 68(2): 159-170. DOI:10.1002/maco.201608831
[22] TAN C L, ZHOU K S, MA W Y, et al. Microstructural evolution, nanoprecipitation behavior and mechanical properties of selective laser melted high-performance grade 300 maraging steel[J]. Materials & Design, 2017, 134: 23-34. DOI:10.1016/j.matdes.2017.08.026
[23] BASAK A, DAS S. Microstructure of nickel-base superalloy MAR-M247 additively manufactured through scanning laser epitaxy (SLE)[J]. Journal of Alloys and Compounds, 2017, 705: 806-816. DOI:10.1016/j.jallcom.2017.02.013
[24] SCHNEIDER J, LUND B, FULLEN M. Effect of heat treatment variations on the mechanical properties of Inconel 718 selective laser melted specimens[J]. Additive Manufacturing, 2018, 21: 248-254. DOI:10.1016/j.addma.2018.03.005
[25] ZHOU L B, YUAN T C, Li R D, et al. Microstructure and mechanical properties of selective laser melted biomaterial Ti-13Nb-13Zr compared to hot-forging[J]. Materials Science and Engineering A, 2018, 725: 329-340. DOI:10.1016/j.msea.2018.04.001
[26] GIACCHI J V, MORANDO C N, FORNARO O, et al. Microstructural characterization of as-cast biocompatible Co-Cr-Mo alloys[J]. Materials Characterization, 2011, 62(1): 53-61. DOI:10.1016/j.matchar.2010.10.011
[27] KREITCBERG A, BRAILOVSKI V, TURENNE S. Effect of heat treatment and hot isostatic pressing on the microstructure and mechanical properties of Inconel 625 alloy processed by laser powder bed fusion[J]. Materials Science and Engineering A, 2017, 689: 1-10. DOI:10.1016/j.msea.2017.02.038
[28] ZHU L, XU Z F, LIU P, et al. Effect of processing parameters on microstructure of laser solid forming Inconel 718 superalloy[J]. Optics & Laser Technology, 2018, 98: 409-415. DOI:10.1016/j.optlastec.2017.08.027
[29] HARRISON N J, TODD I, MUMTAZ K. Reduction of micro-cracking in nickel superalloys processed by selective laser melting: a fundamental alloy design approach[J]. Acta Materialia, 2015, 94: 59-68. DOI:10.1016/j.actamat.2015.04.035
[30] GHOSH S, OFORI-OPOKU N, GUYER J E. Simulation and analysis of γ-Ni cellular growth during laser powder deposition of Ni-based superalloys[J]. Computational Materials Science, 2018, 144: 256-264. DOI:10.1016/j.commatsci.2017.12.037
[31] FESHARAKI M N, SHOJA-RAZAVI R, MANSOURI H A, et al. Evaluation of the hot corrosion behavior of Inconel 625 coatings on the Inconel 738 substrate by laser and TIG cladding techniques[J]. Optics & Laser Technology, 2019, 111: 744-753. DOI:10.1016/j.optlastec.2018.09.011
[32] LIU H F, TAN C K I, WEI Y F, et al. Laser-cladding and interface evolutions of inconel 625 alloy on low alloy steel substrate upon heat and chemical treatments[J]. Surface and Coatings Technology, 2020, 404: 126607. DOI:10.1016/j.surfcoat.2020.126607
[33] TUOMINEN J, VUORISTO P, M?NTYL? T, et al. Microstructure and corrosion behavior of high power diode laser deposited Inconel 625 coatings[J]. Journal of Laser Applications, 2003, 15(1): 55-61. DOI:10.2351/1.1536652
[34] 陈秀娟, 赵国瑞, 董东东, 等. 激光选区熔化制造Inconel 625高温合金的组织和力学性能[J]. 中国激光, 2019, 46(12): 70-78.
CHEN Xiujuan, ZHAO Guorui, DONG Dongdong, et al. Microstructure and mechanical properties of inconel 625 superalloy fabricated by selective laser melting[J]. Chinese Journal of Lasers, 2019, 46(12): 70-78. DOI:10.3788/CJL201946.1202002
[35] LI W, YANG Y, LIU J, et al. Enhanced nanohardness and new insights into texture evolution and phase transformation of TiAl/TiB2 in-situ metal matrix composites prepared via selective laser melting[J]. Acta Materialia, 2017, 136: 90-104. DOI:10.1016/j.actamat.2017.07.003
[36] YADROITSEV I, KRAKHMALEV P, YADROITSAVA I, et al. Energy input effect on morphology and microstructure of selective laser melting single track from metallic powder[J]. Journal of Materials Processing Technology, 2013, 213(4): 606-613. DOI:10.1016/j.jmatprotec.2012.11.014
[37] KAMAYA M. Measurement of local plastic strain distribution of stainless steel by electron backscatter diffraction[J]. Materials Characterization, 2009, 60(2): 125-132. DOI:10.1016/j.matchar.2008.07.010
[38] 贺可太, 周柳, 杨乐昌. 选区激光熔化中316L不锈钢的组织与力学性能[J]. 激光与光电子学进展, 2020, 57(9): 123-133.
HE Ketai, ZHOU Liu, YANG Lechang. Microstructure and mechanical properties of 316L stainless steel in the selective laser melting[J]. Laser & Optoelectronics Progress, 2020, 57(9): 123-133. DOI:10.3788/LOP57.091404
[39] 王忠堂, 张晓宇, 邓永刚, 等. 挤压IN690管材的孪晶组织及织构[J]. 稀有金属材料与工程, 2014, 43(9): 2252-2256.
WANG Zhongtang, ZHANG Xiaoyu, DENG Yonggang, et al. Twins and texture of super-alloy In690 tube by extrusion[J]. Rare Metal Materials and Engineering, 2014, 43(9): 2252-2256.
[40] DESGRANGES C, LEQUIEN F, AUBLANT E, et al. Depletion and voids formation in the substrate during high temperature oxidation of Ni-Cr alloys[J]. Oxidation of Metals, 2013, 79(1-2): 93-105. DOI:10.1007/s11085-012-9328-0
[41] SAEIDI K, GAO X, ZHONG Y, et al. Hardened austenite steel with columnar sub-grain structure formed by laser melting[J]. Materials Science and Engineering A, 2015, 625(feb.11): 221-229. DOI:10.1016/j.msea.2014.12.018