Huandi Huang1, Jie Ding1, Xianshu Liu1, Guojun Xie2,Dihui Song1
(1.State Key Laboratory of Urban Water Resource and Environment, Harbin Institute of Technology, Harbin 150090, China;2.Advanced Water Management Centre,The University of Queensland,Brisbane 4072,Australia)
Abstract:
Due to its persistence and bio-toxicity, benzothiazole (BTH) cannot be biodegraded efficiently. Recent work has shown that removal rates of biorefractory organics can be enhanced by the addition of co-substrates. In this work, ethanol, acetate, propionate and butyrate were added as co-substrates in order to promote the degradation of BTH in microbial electrolysis cell (MEC). By probing the changes in degradation rates of BTH in the presence of different co-substrates, it was observed that all the four co-substrates can enhance the BTH degradation in MEC, both the efficiency (EBTH) and the rate (RBTH). It was also found that acetate is more effective than others, which made the degradation efficiency of BTH up to 90% with acetate-C at 350 mg/L (measuring by the carbon content of co-substrate, the same below),within 6 h and the degradation rate of BTH arrived 0.001 2/(mg·h). The microbacteria in MEC have also been influenced by different co-substrates. This metabolism of the co-substrates enables the microbacteria on anode to generate ATP and thus grow to ensure the microbacteria activity. Therefore, this work showed that the addition of co-substrates such as acetate can be a novel and efficient approach for improving the elimination of BTH from wastewaters by MEC system.
Key words: MEC benzothiazole co-substrate acetate
DOI:10.11916/j.issn.1005-9113.2016.05.009
Clc Number:X592
Fund:
Huandi Huandi, Jie Ding, Xianshu Liu, Guojun Xie, Dihui Song. Effect of Co-substrate on Degradation of Benzothiazole in MEC[J]. Journal of Harbin Institute of Technology, 2016, 23(5): 62-68. DOI: 10.11916/j.issn.1005-9113.2016.05.009.

Fund Sponsored by the National Water Pollution Control and Management Technology Major Projects (Grant No.2013ZX07201007), the Program for New Century Excellent Talents in University (Grant No. NCET-11-0795) and the State Key Laboratory of Urban Water Resource and Environment (Grant No.2012DX04) Corresponding author Jie Ding, E-mail:dingjie123@hit.edu.cn Article history Received: 2015-05-27
Contents Abstract Full text Figures/Tables PDF
Effect of Co-substrate on Degradation of Benzothiazole in MEC
Huandi Huandi1, Jie Ding1


1. State Key Laboratory of Urban Water Resource and Environment, Harbin Institute of Technology, Harbin 150090, China;
2. Advanced Water Management Centre, The University of Queensland, Brisbane 4072, Australia
Received: 2015-05-27
fund: Sponsored by the National Water Pollution Control and Management Technology Major Projects (Grant No.2013ZX07201007), the Program for New Century Excellent Talents in University (Grant No. NCET-11-0795) and the State Key Laboratory of Urban Water Resource and Environment (Grant No.2012DX04)
Corresponding author: Jie Ding, E-mail:dingjie123@hit.edu.cn
Abstract: Due to its persistence and bio-toxicity, benzothiazole (BTH) cannot be biodegraded efficiently. Recent work has shown that removal rates of biorefractory organics can be enhanced by the addition of co-substrates. In this work, ethanol, acetate, propionate and butyrate were added as co-substrates in order to promote the degradation of BTH in microbial electrolysis cell (MEC). By probing the changes in degradation rates of BTH in the presence of different co-substrates, it was observed that all the four co-substrates can enhance the BTH degradation in MEC, both the efficiency (EBTH) and the rate (RBTH). It was also found that acetate is more effective than others, which made the degradation efficiency of BTH up to 90% with acetate-C at 350 mg/L (measuring by the carbon content of co-substrate, the same below), within 6 h and the degradation rate of BTH arrived 0.001 2/(mg·h). The microbacteria in MEC have also been influenced by different co-substrates. This metabolism of the co-substrates enables the microbacteria on anode to generate ATP and thus grow to ensure the microbacteria activity. Therefore, this work showed that the addition of co-substrates such as acetate can be a novel and efficient approach for improving the elimination of BTH from wastewaters by MEC system.
Key words: MEC benzothiazole co-substrate acetate
1 IntroductionBenzothiazole (BTH), as a nitrogen-heterocyclic compound, has been widely used in the manufacture of pesticides[1], pharmacy[2] and industry[3] because of its extensive biological inhibition to viruses, fungi, and pests. But in the meanwhile, since BTH possesses the properties of water-soluble, poor biodegradability and adsorption capacity, only a little proportion of BTH can be eliminated by conventional wastewater treatment processes. As a result, most of BTH is discharged into the rivers and accumulates in the environment. Studies have demonstrated that BTH has certain toxicity to aquatic organisms. More importantly, its biological accumulation possibly leads carcinogenic effect to human[4]. To reduce exposure and a potential risk posed by BTH for aquatic organisms and human health, water treatment processes for their efficient abatement are needed. The conventional treatments such as H2O2/UV[5], Fenton[6], ozone oxidation[7] could remove BTH in wastewater, but were energy and cost intensive. Electro-assisted biodegradation of BTH in microbial electrolysis cell (MEC) proposed by Liu et al.[8] shed light on a potential cost-effective and high efficient BTH removal process. Such a process has potential advantages in terms of versatility, environmental compatibility, and no chemical usage[9].
The applications of MECs were mainly focused on the production of hydrogen in the past decades[10-11]. Its application in wastewater treatment appeared gradually in recent years, especially for refractory wastewater. Ren[12] studied the degradation of chlorinated nitrobenzene in MEC, and the degradation efficiency got to 90%. Xu et al.[13] investigated the degradation of Pure Terephthalic Acid (PTA) in microbial electrolysis cell, and the degradation efficiency of PTA reached up to 92%. At present, it has been confirmed MEC can strengthen a variety of anaerobic degradation of organic pollutants, including fragrance hydrocarbon[14], 1, 2-dichloethane[15], toluene[16], nitrobenzene[17], azo dye[18] and diclofenac[19].
In the MEC, under the synergy of microbacteria and electricity, the benzothiazole firstly occurred with hydroxyl to generate 2-hydroxy benzothiazole, after that the C=N bond broken to complete the process of detoxification of BTH. Up to date, many studies showed that the addition of co-substrates (an easily degradable substrate) such as glucose, sucrose, methanol and propionate[20-21] could enhance the degradation rates. The addition of co-substrates promoted the BTH degradation in MEC, and the mechanism roughly included the following three aspects: 1) the co-substrates could act as an inducing agent for biodegradative enzymes; 2) these substrates provided sufficient carbon source and energy for electrochemically active microbacteria, and the macrobacteria constantly proliferated and maintained highly active; 3) the energy produced in the utilization of the co-substrate promoted the nitrogen heterocyclic cracking.
Despite of many reports on the co-substrate used in MEC were made, a comprehensive comparison on the various co-substrates, which had been used and can possibly be used in MEC, is still lacking. Besides, how the co-substrate influence the degradation of BTH in MEC has not been reported. The main purpose of this study was to investigate the effects of the types of co-substrate as well as the initial concentration of co-substrate on BTH removal in order to provide theoretical basis for effectively degrading BTH in MEC. The dynamics of the BTH degradation with addition of different co-substrates were also explored in order to determine the relationship between its degradation rates and the concentration of co-substrate. The microbacteria were also evaluated by the Single-Strand Conformation Polymorphism (SSCP) technology.
2 Materials and Methods2.1 Experiment MaterialThis research was carried out in a round single chamber MEC (as show in Fig. 1), made of plexiglass. The height was 150 mm and the inner diameter was 90 mm with a working volume of 1 000 mL.The anode and cathode (distance 40 mm) of the reactor were composed by carbon paper (100 mm×50 mm), and the gap was filled with graphite granules. The graphite granules could provide major active sites for microbacteria to adhere to, and also promote electrons transfer. Both anode and cathode were equipped with an Ag/AgCl reference electrode (+0.2 V vs. NHE) to measure the electrode potential. The power source supplies a steady voltage of 0.7 V between anode and cathode.
Figure 1
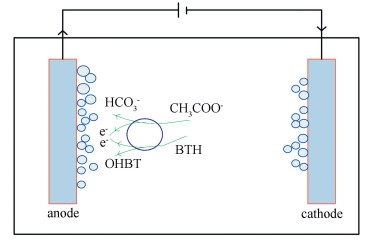
2.2 Inoculation and StartupSewage sludge to inoculate the reactors was collected from the secondary settling tank in a wastewater treatment plant for municipal sewage in Harbin, China. The sludge was kept in anaerobic condition for 48 h, and then was mixed with electrode medium (50/50, volume ratio), finally was inoculated into the MEC reactors. After inoculation, the reactors were operated at a fixed voltage of 0.7 V immediately for startup. The medium was close to the outflow of pharmaceutical wastewater, and the concentration of BTH was 50 mg/L. Nitrogen source and phosphorus source were put in the reactor with a proportion COD:N:P=200:5:1. The reactor was buffered by using phosphate buffer solutions (KH2PO4 and K2HPO4, 50 mmol/L, pH 7.0).
2.3 ExperimentIn this experiment, four reactors fed with ethanol, acetate, propionate and butyrate were set up. After startup was finished, experiments were carried out through analysis of BTH concentration under a fixed applied voltage of 0.7 V. The medium in electrode chamber was replaced at the end of each cycle. Reactors were flushed with dinitrogen gas (99.9%) for 20 min to remove oxygen in the solution and headspace. To study the effect of co-substrate concentration, a series of co-substrate concentrations changed from 0 to 2 400 mg/L stepwise. The same operation of each concentration was repeated seven days to allow steady-stable conditions. The microbacteria samples were taken from anode biofilm in reactors after the tests finished.
2.4 AnalysisThe applied voltage in the study was all supplied by a simple direct current power supply (PS-B202D, Yizhan Electronic Instrument Co., Ltd.). The concentrations of BTH and intermedia 2-hydroxy-benzothiazole (OHBT) in samples were determined by a HPLC (Perkin Elmer Series 200, USA) equipped with a C18 column (Phenomenex, USA). The mobile phase was methanol/water (55:45, volume ratio) at a flow rate of 1 mL/min, injection volume was 10 μL. Detection of the samples was made at a wavelength of 254 nm. All the experiments were done at room temperature of (25±5) ℃. The microbacteria were evaluated using the Single-Strand Conformation Polymorphism (SSCP) technology.
3 Results and Discussions3.1 Effect of Different Co-substrates on the Degradation Rates of BTHTo obtain a better understanding about the effect of different co-substrates as well as the initial concentration of co-substrate on BTH removal in MEC, four reactors with addition of ethanol, acetate, propionate and butyrate were set up. In the study, only a small amount of the intermediate OHBT by HPLC were detected within 0.5 h in the process of continuous sample but have a large removal of BTH in the reactors. This showed that BTH adsorption to the biofilm occupied a dominant role within 0.5 h. And about 40% of BTH concentration was absorbed to the biofilm within 0.5 h (the initial concentration of BTH was 50 mg/L, and about 30 mg/L was detected after 0.5 h), and then the degradation of BTH played a major role (Fig. 2). At the beginning 0.5 h, due to the effect of the biofilm adsorption, the BTH concentration decreased quickly. After a period of time, the BTH concentration decreased slowly just because the degradation of BTH achieved a balance of adsorption and desorption. The similar phenomenon was also found in the study of Zhang[22]. He thought the ingot blue concentrations decrease quickly at the beginning of the reaction was resulted by the adsorption of the sludge.
Figure 2
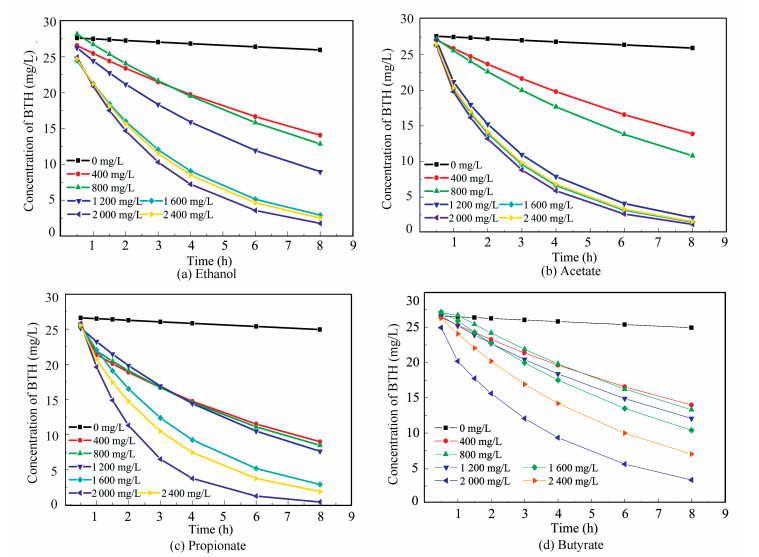
The addition of co-substrates caused an increase in the degradation of BTH in wastewater compared to the control in the absence of any co-substrates. The RBTH could increase 13% in MEC than in a simple bioreactor with the same co-substrate in the absence of electrode with the same co-substrates in our pre-research[23]. The degradation rates were higher as the co-substrates were added into the reactors. The degradation of BTH in MEC was in conformity to pseudo-first-order kinetic model. So the first-order kinetics constant was used to stand for the degradation rate. For instance, the RBTH improved from 0.022/h to 0.405 3/h with acetate-C at 350 mg/L (Fig. 2). To compare the RBTH with addition of different co-substrates, the RBTH were converted in terms of co-substrates equivalents. The RBTH was higher as the co-substrates were added into the reactors. For example, when acetate was added in the reactor, the RBTH in equivalent was 0.001 2 /(mg·h).This is 1.7-fold higher than that observed with propionate-C (0.000 7 /(mg·h)) at 750 mg/L (Table 1). This is also 2.4-fold higher than that obtained with ethanol and 4-fold higher than that observed with butyrate, respectively. These results indicated that acetate was better co-substrates for improving the degradation of BTH in the reactor. All the co-substrates enhanced the degradation of BTH indicating that the co-substrate served as carbon (nutrient) and fuel sources for the metabolism of microbacteria. It has been reported that the readily oxidizable carbon sources act as inducing agents of oxidizing enzymes or by providing reducing power for the degradation of recalcitrant organic compounds[14, 16, 24].Huang et al.[20] demonstrated that pentachlorophenol (PCP) was more rapidly degraded in MEC with acetate as co-substrates than in open circuit controls, with degradation rates of (0.12±0.01) mg/(L·h) in acetate-fed at an initial PCP concentration of 15 mg/L. Martínez et al.[25] also suggested that the addition of co-substrates such as acetate could be a better alternative for improving the elimination of chlorophenols from wastewater by denitrifying sludge. The higher RBTH was detected with acetate among all the four co-substrates, followed by propionate. The similar RBTH were found with butyrate and ethanol as co-substrates but lower than the former. It was considered that acetate[26]could be used by the bacteria on anode directly; while ethanol[27-28]and butyrate[29] were utilized mainly by exchanging to substrates easier to uptake; differently, propionate[30] could be used by the bacteria on anode directly or indirectly in the same system.
表 1
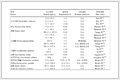
None-ln(ρt/ρ0)=-0.022 0t-0.499 1 0.022 0-
Ethanol700ln(ρt/ρ0)=-0.353 8t-0.519 0 0.353 80.000 5
Acetate350ln(ρt/ρ0)=-0.405 3t-0.507 80.405 30.001 2
Propionate750ln(ρt/ρ0)=-0.550 5t-0.486 2 0.550 50.000 7
Butyrate870ln(ρt/ρ0)=-0.256 6t-0.650 8 0.256 60.000 3
Table 1 Degradation rate of BTH in MEC with addition of co-substrates
1.Amount refers to the carbon content of co-substrates with the most optimal BTH degradation rate; 2.ρt refers to the concentration of BTH at time t; ρ0 refers to the initial concentration; 3.Calculated BTH degradation rate under per mg C of co-substrates equivalent.
The microbial anode potential, which would be lower when substrate was added[31], therefore, when different substrates such as ethanol, acetate, propionate or butyrate were added into the system, the microbial anode potential altered; furtherly affected the degradation of BTH. Wang et al.[32] mentioned that higher hydrogen rate was obtained when microbial anode potential went down to -400 mV or even lower, when substrate concentration was less than 50 mg/L on anode, microbial anode potential went up to -300 mV or above.
For the case with acetate and ethanol as co-substrate in MEC, reactions on anode are as follows.
${\rm{C}}{{\rm{H}}_{\rm{3}}}{\rm{CO}}{{\rm{O}}^{\rm{ - }}}{\rm{ + 4}}{{\rm{H}}_{\rm{2}}}{\rm{O}} \to {\rm{2HCO}}_3^ - + {\rm{9}}{{\rm{H}}^{\rm{ + }}}{\rm{ + 8}}{{\rm{e}}^ - }{E_1} = - 277{\rm{mV}}$ (1)
${\rm{C}}{{\rm{H}}_{\rm{3}}}{\rm{C}}{{\rm{H}}_{\rm{2}}}{{\rm{O}}^{\rm{ - }}}{\rm{ + 5}}{{\rm{H}}_{\rm{2}}}{\rm{O}} \to {\rm{2HCO}}_3^ - + {\rm{13}}{{\rm{H}}^{\rm{ + }}}{\rm{ + 12}}{{\rm{e}}^ - }{E_2} = - 314{\rm{mV}}$ (2)
These two reactions hint that the microbacteria on biofilm gets more energy from the acetate oxidation[33], and then the microbial anode potential gets lower, the power density of the whole circuit could get higher to ensure a quicker degradation rate of BTH. Acetate is a substrate that is more easily oxidized than ethanol, and this might be the reason why the maximum degradation rates of BTH was obtained using acetate as the co-substrate. Martínez et al.[34] also observed a similar role of acetate for toluene mineralization by denitrification, suggesting that acetate could act through enhancing the growth of bacteria populations and as a biochemical enhancer. It is often reported that acetate is the most widespread used co-substrate and has an obvious performance in bio-electrochemical systems (BESs). Martínez et al.[25] also found that when acetate was added, the specific consumption rate of 2-chlorophenol (2-CP) increased 9.0 times. It is calculated that the degradation rate of BTH improved 16-fold with acetate as co-substrate than the control in our study. These results in this work suggested that acetate be the optimum co-substrate for the degradation of BTH in MEC system; further studies are required for a better understanding of the effects of co-substrates on degradation of BTH.
3.2 Effect of Different Concentrations of Co-substrates on the Removal Efficiency of BTHAs shown in Fig. 2, significant removal of BTH was obtained after 6 h in all tests (Fig. 2). Therefore, to compare the effect of the concentrations of co-substrates, the degradation efficiencies of BTH were obtained at 6 h in all reactors with initial BTH concentration of 50 mg/L (Fig. 3).
Figure 3
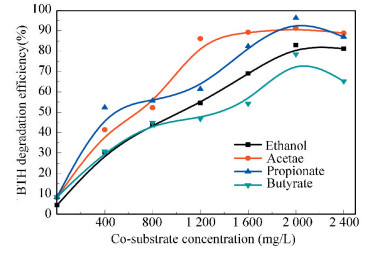
It was shown that all the co-substrates could increase the EBTH in wastewater compared to the control (only 6% of the BTH concentration was removed without addition of any co-substrates). As shown in Fig. 3, the EBTH increased with the increase of the co-substrate concentration with an initial concentration of 50 mg/L. At acetate concentration ranging from 400 mg/L to 1 200 mg/L, and the EBTH increased from 40% to 90%. In the same conditions, it can only increase from 30% to 45% with butyrate as co-substrates. At the same co-substrate concentration of 1 200 mg/L, the EBTH was two-fold with acetate than that observed with butyrate. These results also indicated that acetate was better co-substrate for enhancing the degradation of BTH in MEC. Liu[23] reported that glucose could be used as growth co-substrate for the degradation of BTH. It needed 24 h with glucose as co-substrate to achieve EBTH of 90%. However, it could shorten to 6 h with acetate as co-substrate to achieve the same degradation efficiency. It was observed that acetate as co-substrate was preferable to glucose, as glucose need hydrolyze and acidify into substrates that are easier to metabolize like acetate. Degradation of particulate and colloidal substrates is often rate limiting, as hydrolytic and acidified processes are usually required to covert particulate and colloidal substrates to those substrates that easy to metabolize[35]. It was reported that very high coulombic efficiencies have been achieved with microbial fuel cells for acetate. These higher coulombic efficiencies were reported because the anodic food web does not need to support hydrolysis and acidification of high-molecular-weight constituents compared to anodic food webs in MFCs treating complex organics in wastewater. Electrochemically active microbacteria can readily ingest and oxidize the simple organic substrates, regenerate reducing equivalents with anaerobic respiration processes, and divert electrons to the anode electrode[36-37]. Thus, aside from meeting cell maintenance and growth energy requirements, the microbacteria can efficiently convert the simple organic substrates into electricity. This will contribute to a less complex food web, lower biomass concentrations, and a higher concentration of electrochemically active microbacteria. We can enforce such an efficient MEC by outsourcing the rate-limiting hydrolysis and acidification steps into a pre-acidification tank. Wastewater pre-acidification is a process commonly used to accelerate soluble organic constituent conversion. It may be advantageous to have some or most of the fermentation occurs in a pre-acidification tank that precedes the MEC. In this case, the MEC receives simpler organic compounds that can be oxidized more directly by electrochemically active microbacteria, thereby simplifying the structure and function of the electrochemically active microbacteria community in the biofilm in anode and helping bring about higher current density[38].
This provided a guiding significance on controlling the extent that the upstream arrived. The residence time in pre-acidification tanks is typically short enough to prevent methanogenesis and to only promote formation of substrates that is easy to metabolize (acetate, butyrate, and propionate, ethanol) from anaerobic microbial conversion of soluble organic substrates, such as sugars and proteins. Further studies are required for a better understanding of the process how the soluble organic substrates transform to substrates that are easy to metabolize.
3.3 Analysis of Microbacteria in MECThe microbacteria on anode contributes to electron transport and anode potential, further affects the MEC performance[39]. And anodic microbacteria have been extensively studied recently. Previous analyses of microbacteria on anode in MEC have revealed a high diversity. The most widely described species are Geobacter and Shewanella, which are acknowledged as functional bacteria with extracellular electron transfer in MEC[40]. In the meanwhile, Proteobacteria and Firmicutes are found at high abundancy on anode recently[41]. Different co-substrates influenced the integral composition of the microbacteria on the anodic biofilm, and then influenced the rate of oxidizing co-substrates, finally resulted in the different rate of electron transport and the microbacteria acitivity. All the processes further determined the BTH degradation. The microbacteria were evaluated by SSCP (Fig. 4).
Figure 4
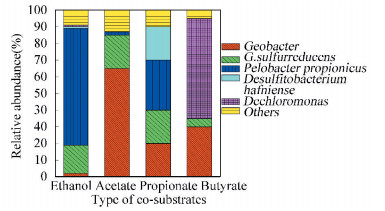
Anaerobic co-metabolism of BTH is mainly based on oxidation on anode, in which BTH serves as an electron donor and the co-substrate (an easily degradable substrate) is used to reduce the toxicity and growth inhibition of BTH to microbacteria. This metabolism of the co-substrates enables the microbacteria on anode to generate ATP and thus grow to ensure the microbacteria acitivity. No matter the type and amount of microbacteria, the reactors with suitable co-substrate were abundant than the control. The microbacteria in the control were killed because of the toxicity of BTH as time went on. Geobacter, firstly confirmed the presence in MEC by Parameswaran et al.[42], was detected in all reactors.
Geobacter dominated MEC with acetate as co-substrate and it could transport electron at the highest speed. G.sulfurreducens was less abundant than Geobacter but essential. G. sulfurreducens can produce electric conductivity nanowires, and then adhere to the electrode surface to grow and remain active for a long time in order to transfer electron to electrode in the process of oxidation of organic substrates. A higher power density and coulomb efficiency could occur with its appearance[43].The main microbacteria were Pelobacter propionicus and G. sulfurreducens with propionate as co-substrate. Pelobacter propionicus converted propionate to acetic acid and G.sulfurreducens transport electron produced by acetic acid oxidation process. Butyrate and ethanol needed transform to substrate easier to utilize by microbacteria. In the community of butyrate as co-substrate, Dechloromonas and Geobacter were more abundant than others[30]. It was assumed that butyrate was converted into acetic acid by Dechloromonas, and acetic acid then was utilized by Geobacter. And the low concentration of acetic acid was detected in the process[29]. While in the reactor with ethanol as co-substrate in MEC, acetic acid was generated by Pelobacter propionicus, which can produce and secrete soluble quinones to carry out the extracellular process[44].
In this study, we focused on microbacteria characterization when a variety of simple substrates, such as ethanol, acetate, propionate and butyrate existed simultaneously. Nevertheless, the BTH degradation and microbacteria structure in MEC have not been revealed in the presence of volatile fatty acid mixtures such as acetate and propionate in different proportions. Thus, the performance and structure of anode bacteria communities in MECs operated at different initial concentrations of a mixture of volatile fatty acids need to be further explored.
4 ConclusionsThe addition of co-substrate enhanced the degradation of BTH in MEC, increasing both the degradation rate and removal efficiency compared with the control. It was also observed that acetate was better co-substrate than others due to its relatively easy oxidation. It was also found that acetate is more effective than others, which makes the EBTH up to 90% with acetate-C at 350 mg/L within 6 h and the RBTH reached 0.001 2/(mg·h). The analysis of microbacteria in MEC indicated that the reactor with suitable co-substrate is abundant, no matter the type and amount of microbacteria. The type and abundance of microbacteria were different with different co-substrate. These results contributed to a better understanding on the BTH degradation in MEC and were also useful for suitable proposals for degrading BTH in wastewater.
References
[1]Hong Y P, Song B A, Wu P, et al. A review on benzothiazole pesticide biological activity.Anhui Agricultural Science, 2005(7): 1254-1257.(

[2]Sharma P C, Sinhmar A, Sharma A, et al. Medicinal significance of benzothiazole scaffold: An insight view.Journal of Enzyme Inhibition and Medicinal Chemistry, 2013, 28(2): 240-266.DOI:10.3109/14756366.2012.720572(

[3]Luo J, Cai C, Lv C X, et al. A rieview on microwave organic synthetic chemistry.The Synthesis Chemistry, 2002(1): 17-24.(

[4]Wang J C, Xiong L, Zhang H J, et al. Solid phase extraction-HPLC for determination of benzotriazole and benzothiazole in surface water.Chromatography, 2013(2): 139-142.(

[5]Andreozzi R, Caprio V, Marotta R. Oxidation of benzothiazole, 2-mercaptobenzothiazole and 2-hydroxybenzothiazole in aqueous solution by means of H2O2/UV or photoassisted Fenton systems.Journal of Chemical Technology & Biotechnology, 2001, 76(2): 196-202.(

[6]Sarasa J, Llabres T, Ormad P, et al. Characterization and photo-Fenton treatment of used tires leachate.Journal of Hazardous Materials, 2006, 136(3): 874-881.DOI:10.1016/j.jhazmat.2006.01.023(

[7]Derco J, Melicher M, Kassai A, et al. Removal of benzothiazoles by ozone pretreatment.Environmental Engineering Science, 2011, 28(11): 781-785.DOI:10.1089/ees.2010.0399(

[8]Liu C M, Ding J, Liu X S, et al. Degradation of Benzothiazole in electro-assisted microbial reactor.Environmental Science, 2014(11): 4192-4197.(

[9]Kong F, Wang A, Ren H. Improved 4-chlorophenol dechlorination at biocathode in bioelectrochemical system using optimized modular cathode design with composite stainless steel and carbon-based materials.Bioresource Technology, 2014, 166: 252-258.DOI:10.1016/j.biortech.2014.05.049(

[10] Bai Y X, Li X H, Fan Y T, et al. The analysis of in the efficient production and process with glucose in microbial cell (MEC). The 29th Academic Conference in the Chinese Chemical Society.Beijing, 2014.1-8. (

[11]Montpart N, Rago L, Baeza J A, et al. Hydrogen production in single chamber microbial electrolysis cells with different complex substrates.Water Research, 2015, 68: 601-615.DOI:10.1016/j.watres.2014.10.026(

[12] Ren L Y. The Startup Countermeasure of Microbial Electrolysis Cell to Degradate of Chlorinated Nitrobenzene. Harbin:Harbin Institute of Technology, 2013. (

[13]Xu Y, Wang L Y, Jiang Y Y, et al. The reasearch on PTA wastewater treatment and synchronous hydrogen production in MEC.Journal of Solar Energy, 2014(4): 716-720.(

[14]Wang S J, Loh K C. New cell growth pattern on mixed substrates and substrate utilization in cometabolic transformatin of 4-chlorophenol.Water Research, 2000, 34(15): 3786-3794.DOI:10.1016/S0043-1354(00)00144-5(

[15]Zhang J, Slevin C J, Murtom?ki L, et al. Microelectrochemical measurements at expanding droplets:Effect of surfactant adsorption on electron transfer kinetics at liquid/liquid interfaces.Langmuir, 2001, 17(3): 821-827.DOI:10.1021/la001113z(

[16]Martínez-Hernández S, Olguín E J, Gómez J, et al. Acetate enhances the specific consumption rate of Toluene under denitrifying conditions.Archives of Environmental Contamination and Toxicology, 2009, 57(4): 679-687.DOI:10.1007/s00244-009-9321-z(

[17]Zhang J, Zhang Y, Quan X. Bio-electrochemical enhancement of anaerobic reduction of nitrobenzene and its effects on microbial community.Biochemical Engineering Journal, 2015, 94: 85-91.DOI:10.1016/j.bej.2014.11.018(

[18]Wang Y, Wang A, Liu W, et al. Enhanced azo dye removal through anode biofilm acclimation to toxicity in single-chamber biocatalyzed electrolysis system.Bioresource Technology, 2013, 142: 688-692.DOI:10.1016/j.biortech.2013.05.007(

[19]Gusseme B D, Soetaert M, Hennebel T, et al. Catalytic dechlorination of diclofenac by biogenic palladium in a microbial electrolysis cell.Microbial Biotechnology, 2012, 5(3): 396-402.DOI:10.1111/mbt.2012.5.issue-3(

[20]Huang L, Gan L, Zhao Q, et al. Degradation of pentachlorophenol with the presence of fermentable and non-fermentable co-substrates in a microbial fuel cell.Bioresource Technology, 2011, 102(19): 8762-8768.DOI:10.1016/j.biortech.2011.07.063(

[21]Majumder P S, Gupta S K. Effect of carbon sources and shock loading on the removal of chlorophenols in sequential anaerobic-aerobic reactors.Bioresource Technology, 2008, 99(8): 2930-2937.DOI:10.1016/j.biortech.2007.06.024(

[22] Zhang W. Different Substrates Metabolic Degradation of Indigo Blue in Waste Water. Guangzhou:Guangzhou University of Technology, 2013. (

[23] Liu C M. MEC Reactor to Remove Benzothiazole in Antibiotics Wastewater. Harbin:Harbin Institute of Technology, 2014. (

[24]Dietrich G W J. Anaerobic degradation of chlorophenol by an enrichment culture.Applied Microbiology and Biotechnology, 1990, 34(2): 253-258.DOI:10.1007/BF00166791(

[25]Martínez-Gutiérrez E, González-Márquez H, Martínez-Hernández S, et al. Effect of phenol and acetate addition on 2-chlorophenol consumption by a denitrifying sludge.Environmental Technology, 2012, 33(12): 1375-1382.DOI:10.1080/09593330.2011.627882(

[26]Call D F, Wagner R C, Logan B E. Hydrogen production by geobacter species and a mixed consortium in a microbial electrolysis cell.Applied and Environmental Microbiology, 2009, 75(24): 7579-7587.DOI:10.1128/AEM.01760-09(

[27]Kiely P D, Rader G, Regan J M, et al. Long-term cathode performance and the microbial communities that develop in microbial fuel cells fed different fermentation endproducts.Bioresource Technology, 2011, 102(1): 361-366.DOI:10.1016/j.biortech.2010.05.017(

[28]Parameswaran P, Zhang H, Torres C S I, et al. Microbial community structure in a biofilm anode fed with a fermentable substrate:The significance of hydrogen scavengers.Biotechnology and Bioengineering, 2010, 105(1): 69-78.DOI:10.1002/bit.v105:1(

[29]Liu H, Cheng S, Logan B E. Production of electricity from acetate or butyrate using a single-chamber microbial fuel cell.Environmental Science & Technology, 2005, 39(2): 658-662.(

[30]Chae K, Choi M, Lee J, et al. Effect of different substrates on the performance, bacterial diversity, and bacterial viability in microbial fuel cells.Bioresource Technology, 2009, 100(14): 3518-3525.DOI:10.1016/j.biortech.2009.02.065(

[31] Sun D. Directional Selection of Anode Microorganism to Promote Effectiveness of Electrochemical System. Harbin:Harbin Institute of Technology, 2013. (

[32]Wang A J, Liu W Z, Ren N Q, et al. Key factors affecting microbial anode potential in a microbial electrolysis cell for H2 production.International Journal of Hydrogen Energy, 2010, 35(24): 13481-13487.DOI:10.1016/j.ijhydene.2009.11.125(

[33] Wang Y Z. Biological Electrochemical System Enhances Decoloring Azo Dye Acid Black 10B and Action Mechanism. Harbin:Harbin Institute of Technoogy, 2014. (

[34]Martínez-Hernández S, Olguín E J, Gómez J, et al. Acetate enhances the specific consumption rate of toluene under denitrifying conditions.Archives of Environmental Contamination and Toxicology, 2009, 57(4): 679-687.DOI:10.1007/s00244-009-9321-z(

[35]Dimock R, Morgenroth E. The influence of particle size on microbial hydrolysis of protein particles in activated sludge.Water Research, 2006, 40(10): 2064-2074.DOI:10.1016/j.watres.2006.03.011(

[36]Gregory K B, Bond D R, Lovley D R. Graphite electrodes as electron donors for anaerobic respiration.Environmental Microbiology, 2004, 6(6): 596-604.DOI:10.1111/emi.2004.6.issue-6(

[37]Lovley D R. The microbe electric:Conversion of organic matter to electricity.Current Opinion in Biotechnology, 2008, 19(6): 564-571.DOI:10.1016/j.copbio.2008.10.005(

[38]Mahmoud M, Parameswaran P, Torres C I, et al. Fermentation pre-treatment of landfill leachate for enhanced electron recovery in a microbial electrolysis cell.Bioresource Technology, 2014, 151: 151-158.DOI:10.1016/j.biortech.2013.10.053(

[39]Nam J, Tokash J C, Logan B E. Comparison of microbial electrolysis cells operated with added voltage or by setting the anode potential.International Journal of Hydrogen Energy, 2011, 36(17): 10550-10556.DOI:10.1016/j.ijhydene.2011.05.148(

[40]Aelterman P, Versichele M, Marzorati M, et al. Loading rate and external resistance control the electricity generation of microbial fuel cells with different three-dimensional anodes.Bioresource Technology, 2008, 99(18): 8895-8902.DOI:10.1016/j.biortech.2008.04.061(

[41]Freguia S, Rabaey K, Yuan Z, et al. Electron and carbon balances in microbial fuel cells reveal temporary bacterial storage behavior during electricity generation.Environmental Science & Technology, 2007, 41(8): 2915-2921.(

[42]Parameswaran P, Zhang H, Torres C S I, et al. Microbial community structure in a biofilm anode fed with a fermentable substrate: The significance of hydrogen scavengers.Biotechnology and Bioengineering, 2010, 105(1): 69-78.DOI:10.1002/bit.v105:1(

[43]Yates M D, Kiely P D, Call D F, et al. Convergent development of anodic bacterial communities in microbial fuel cells.The ISME Journal, 2012, 6(11): 2002-2013.DOI:10.1038/ismej.2012.42(

[44]Wang Y, Masuda M, Tsujimura S, et al. Electrochemical regulation of the end-product profile in Propionibacterium freudenreichii ET-3 with an endogenous mediator.Biotechnology and Bioengineering, 2008, 101(3): 579-586.DOI:10.1002/bit.v101:3(
