Lead sulfide colloidal quantum dots (PbS CQDs), as a novel material for optoelectronic devices, have lately attracted a lot of attention mainly because of their tunable optoelectronic characteristics and low-temperature film preparation. PbS CQDs can harvest infrared (IR) light in single-junction, multi-junction, and tandem solar cells[1-3]. Thanks to the combination of surface chemical engineering and device structure engineering, the power conversion efficiency (PCE) for PbS CQDs solar cells has increased from less than 1% in 2005 to current 13.8%[4, 5].
Exploring and implementing the idea of bulk heterojunction (BHJ) in CQD solar cells (CQDSCs) is intriguing. The goal is to promote charge separation, provide an independent physical path for transporting electrons and holes, respectively, reduce the likelihood of recombination, lengthen free charge carrier lifetime, and increase the device thickness reasonably[6, 7]. In fact, the BHJ structure obtained by phase separation of nano-scale components in thin films was initially used in organic solar cells (Fig. 1(a))[8]. We will discuss the four directions in BHJ CQDSCs as follows.

class="figure_img" id="Figure1"/>
Download
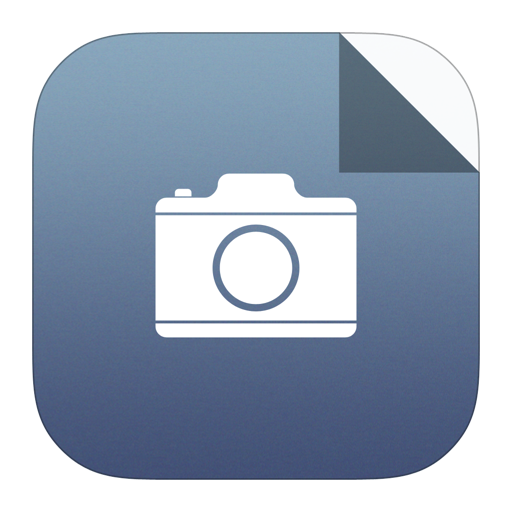
Larger image
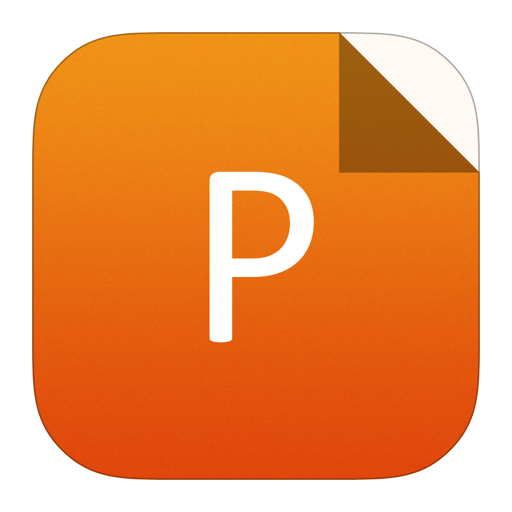
PowerPoint slide
Figure1.
(Color online) (a) Organic bulk-heterojunction (BHJ) solar cells. (b) CQDs/Metal oxides (e.g., ZnO or TiO2) BHJ solar cells. (c) CQDs/Polymer BHJ solar cells. (d) CQDs/Perovskite BHJ solar cells. (e) Schematic of carrier transport in the case of high CQD loading. Reproduced with permission[21], Copyright 2019, Springer Nature. (f) J?V curves of champion devices for BHJ and planar structure under continuous AM 1.5G illumination. Inset is the schematic for monolayer perovskite-bridged CQDs. Reproduced with permission[5], Copyright 2020, Elsevier.
Metal oxides with porous nanostructures (Fig. 1(b)) offer an approach for BHJ-based CQDSCs: CQDs penetrate TiO2 or ZnO mesoporous or nanowire receptors[9, 10]. The infiltrated structure can enhance the interface area, allowing photogenerated electrons to be extracted directly into the nearest TiO2 or ZnO. The BHJ allows the film thickness to reach several micrometers while realizing efficient charge separation and extraction. The short-circuit current density (Jsc) can be significantly improved as high as 30 mA/cm2[11]. However, because of the following two factors, the efficiency does not increase significantly. On one hand, owing to the increase of CQD/metal oxide interface area, BHJ CQDSCs are more susceptible to nonradiative recombination at interfaces because of interfacial defects, leading to larger open-circuit voltage (Voc) loss. On the other hand, the increase in electron extraction rate further exacerbates the imbalance between electron and hole extraction. Thus lower fill factor (FF) and severe hysteresis will appear[12]. Furthermore, building this BHJ structure necessitates a considerable quantity of CQD solution, which is incompatible with CQDSC commercialization. Therefore, using metal oxide nanostructure to create BHJ is not recommended.
Some BHJ structures made of CQD/organic material mixtures have been explored due to their complementary absorption, such as PbS CQD/(P3HT:PCBM)[12], PbSxSe1?x CQD/PDTPBT[13], and PbS CQD/PBDTTT-E-T[14] (Fig. 1(c)). However, there are surface energy difference and chemical mismatch between CQDs and organic materials, making it challenging to realize exciton dissociation at the interfaces in CQD/polymer interpenetration structure, which induces more interface defects. These factors result in much poorer performance for hybrid BHJ CQDSC than that of CQD/organic bilayer device[14]. Current research also shows that the polymers are more suitable for use as hole-transport layer in CQDSCs[15-17].
The fast development of liquid-state ligand exchange (LSLE) method (i.e. CQD ink technologies) in recent years has enhanced CQDSC performance while simplifying the preparation process, allowing PbS CQDs/perovskite BHJ structures to be prepared (Fig. 1(d)). The metal halide perovskite and PbS CQDs have a great lattice match, enabling epitaxial growth of perovskite at CQDs surface. CQDs are effectively passivated by perovskite with a larger bandgap, forming a nano-level Type-I heterojunction (Fig. 1(e)). To construct this interpenetrating structure, in addition to the earliest MAPbI3[18], all-inorganic CsPbI3[19] and tri-cation (CsMAFA)[20] perovskites were also tried. The performance improvement of PbS CQDs/perovskite devices is mainly attributed to the depletion region at the nano-scale heterojunctions, where charge separation occurs quickly and then electron and hole transport through different phase (i.e., CQD phase and perovskite phase), respectively. This leads to reduced nonradiative recombination and enhanced charge collection, thus significant improvement in Jsc and FF. For the BHJ formed by the epitaxial growth, perovskite composition can be adjusted to achieve a lower lattice mismatch between PbS CQDs and perovskite, promoting the formation of a lattice-anchoring (e.g. PbS CQDs/CsPbBrxI3?x)[21]. The lattice-anchoring could improve the stability of perovskite and CQDs simultaneously and significantly increase the carrier mobility of the active layer. Moreover, the transport of free charge carriers among CQDs in this BHJ structure is greatly affected by the thickness of perovskite between two neighboring CQDs. Sargent et al. recently developed a post-processing strategy to form monolayer perovskite bridges among CQDs, which could improve carrier mobility, and increase PCE up to 13.8%. This is the highest efficiency for PbS CQDSCs to date (Fig. 1(f))[5]. The CQDs/perovskite interpenetrating structures formed by epitaxial growth show potential in preparing ideal BHJ CQDSCs. However, it is still challenging to precisely control the lattice matching and perovskite layer thickness among CQDs.
For constructing the BHJ structure, CQDs do not need to be a continuous phase of the same CQDs like organic materials.? When mixing different CQDs to prepare a BHJ structure, if there is enough same CQDs as the neighbor, the BHJ can maintain high carrier mobility (Fig. 2(a)). The prior n-type CQD/p-type CQD BHJ devices used two different QD materials as donors and acceptors, respectively, such as n-type Bi2S3 CQD and p-type PbS CQDs to form the interpenetrating layer[22]. The relative increase in Jsc indicates that nanoscale phase separation promotes charge separation and extraction. However, the lower FF suggests that the solid passivation of different CQDs made by solid-state ligand exchange (SSLE) method is not enough, thus the carrier transport is hindered, preventing these BHJ structures from outperforming bilayer CQDSCs. Considering that the surface ligands of CQDs could be used to tailor CQD energy levels[23], Yang et al. made a homogeneous mixture of the donor (n-type, MAPbI3-PbS CQDs) and acceptor (p-type, thioglycerol-PbS CQDs) with a stable chemical structure, i.e., a Type II CQD BHJ structure (Figs. 2(b) and 2(c)). Thus, the first solar cell based on hybrid quantum-dot ink was developed[24]. The photogenerated electron–hole pairs in CQD active layer with optimized donor : acceptor ratio are effectively separated at the nanoscale interfaces, and the separated electrons and holes can efficiently migrate through respective donor/acceptor phase[24]. Recently, to overcome insufficient passivation of p-type PbS CQDs, Choi et al. developed a cascade surface modification strategy, i.e., re-passivating n-type CQD surfaces with cysteamine (CTA) to obtain a p-type PbS CQD ink with better passivation (Fig. 2(d)). This strategy combines excellent surface passivation and miscibility of the two CQD inks to form a stable mixed QD colloid, which increases carrier diffusion length, improves the charge extraction balance, thus increasing the photosensitive layer thickness. The device PCE can reach 13.3% (Fig. 2(e))[25].

class="figure_img" id="Figure2"/>
Download
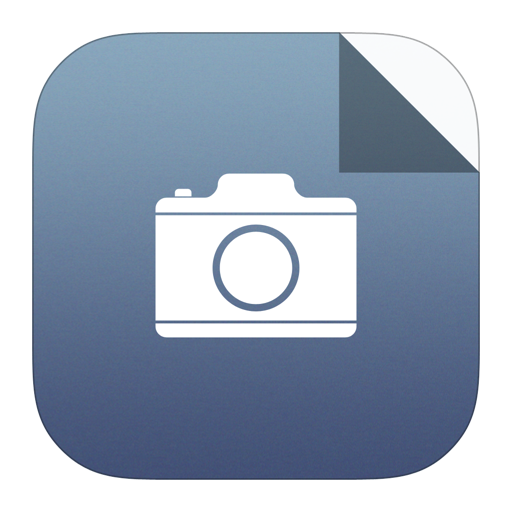
Larger image
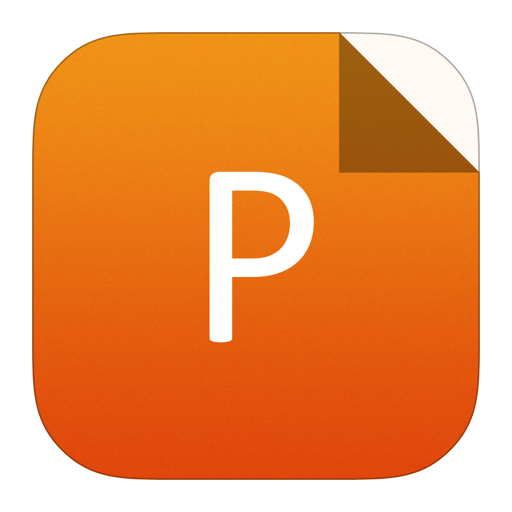
PowerPoint slide
Figure2.
(Color online) (a) n-type CQDs/p-type CQDs BHJ solar cells. (b) Schematic for LSLE process of both n-type and p-type CQDs. (c) Projected density of states demonstrate the offset of band position between MAPbI3- and TG-capped QDs. Reproduced with permission[24], Copyright 2017, Springer Nature. (d) Cascade surface modification (CSM). Step 1: the oleic-acid ligands are exchanged with lead halide anions. Step 2: lead halide anions are re-exchanged with the functional ligands to render p-type character. (e) J?V curves for n-type CQD device, p-type CQD device, and CQD BHJ device with optimum film thickness. Reproduced with permission[25], Copyright 2019, Springer Nature.
In summary, building BHJ structures with superior performance is the most important strategy for CQDSCs. CQD/perovskite and n-CQD/p-CQD BHJ devices are promising. The latter structure can easily improve the PCE through precise tailoring. The second focus is on CQD surface passivation and interface engineering. Novel ligands and passivation methods are desired to improve p-type CQDs' performance and stability. The third focus is on balancing the charge transport.
Acknowledgments
This research was supported by the Japan Science and Technology Agency (JST) Mirai program (JPMJMI17EA), MEXT KAKENHI Grant (26286013, 17H02736). L. Ding thanks the National Key Research and Development Program of China (2017YFA0206600) and the National Natural Science Foundation of China (51773045, 21772030, 51922032 and 21961160720) for financial support.