1.
Introduction
AlN is of great potential in the implantation of deep ultraviolet (DUV) emitters because of its wide bandgap (~6.2 eV), excellent UV transparency and high thermal conductivity[1, 2]. Low threading dislocation density (TDD) is necessary to improve the quantum efficiency of UV light-emitting diodes (LEDs)[3]. Recently, the bulk AlN substrate with a dislocation density (DD) of 103 cm–2 has been achieved[4, 5]. However, the high impurity concentration and high cost has made it inferior to the AlN/sapphire template for commercial motivations. To date, the reported full width at half maximum (FWHM) values of (0002)- and (10



2.
Experiment
In this experiment, AlN layers with a target thickness of 200 nm were deposited on 2-inch c-plane sapphire by radio frequency (RF) reactive magnetron sputtering. High-purity Al (> 99.9999 at%) was used as the target. The RF power was set to 2000 and 3000 W. N2 was used as the sputtering gas in the range of 100–180 sccm, and the substrate temperature was changed from 550 to 700 °C. The chamber background pressure was lower than 3.2 × 10–5 Pa, and the process pressure was varied from 0.31 to 0.55 Pa as the increase of N2 flux. The deposition rate of the sputtered particles was in the range of 0.20–0.35 nm/s, which is mainly determined by RF power[18]. These parameters and characterizations of sputtered AlN are presented in Tables 1 and 2, respectively. Subsequently, the sputtered AlN was annealed in N2 at 1700 °C for 3 h. During the annealing process, the pressure was kept at the atmosphere and the “face to face” method was applied to suppress AlN decomposition[19]. The crystallinity of sputtered and annealed AlN films was investigated by Bede D1 X-ray diffraction (XRD). The FWHM of (0002)- and (10

Sputtering parameter | Value |
Target | Al (> 99.9999 at%) |
Substrate | c-sapphire |
Target to substrate distance | 64.8 mm |
Substrate temperature | 550–700 °C |
N2 flux | 100–190 sccm |
RF power | 2000 and 3000 W |
Process pressure | 0.31 to 0.55 Pa |
Deposition rate | 0.20–0.32 nm/s |
Targeted AlN thickness | 200 nm |
Table1.
Sputtering parameters for the deposition of AlN films.
Table options
-->

Download as CSV
Sputtering parameter | Value |
Target | Al (> 99.9999 at%) |
Substrate | c-sapphire |
Target to substrate distance | 64.8 mm |
Substrate temperature | 550–700 °C |
N2 flux | 100–190 sccm |
RF power | 2000 and 3000 W |
Process pressure | 0.31 to 0.55 Pa |
Deposition rate | 0.20–0.32 nm/s |
Targeted AlN thickness | 200 nm |
Sputtering condition | Deposition rate (nm/s) | Actual thickness (nm) | FWHM values of XRC (arcsec) | RMS (nm) | |
(0002)-plane | (10$ {bar {1}} $2)-plane | ||||
2000 W, 100 sccm, 550 °C | 0.210 | 213.77 | 360 | 3229 | 0.852 |
2000 W, 100 sccm, 600 °C | 0.216 | 219.72 | 270 | 2941 | 1.510 |
2000 W, 100 sccm, 650 °C | 0.233 | 237.42 | 191 | 2714 | 2.200 |
2000 W, 100 sccm, 700 °C | 0.197 | 200.70 | 130 | 2491 | 0.988 |
3000 W, 100 sccm, 550 °C | 0.330 | 207.93 | 184 | 3301 | 0.850 |
3000 W, 100 sccm, 600 °C | 0.340 | 214.19 | 241 | 2923 | 0.995 |
3000 W, 100 sccm, 650 °C | 0.346 | 218.17 | 205 | 2664 | 1.060 |
3000 W, 100 sccm, 700 °C | 0.316 | 199.31 | 119 | 2584 | 0.955 |
2000 W, 150 sccm, 600 °C | 0.206 | 209.28 | 371 | 2776 | 1.860 |
2000 W, 180 sccm, 600 °C | 0.218 | 220.40 | 349 | 2743 | 1.930 |
3000 W, 150 sccm, 600 °C | 0.315 | 198.23 | 292 | 2966 | 1.970 |
3000 W, 180 sccm, 600 °C | 0.322 | 202.65 | 410 | 3049 | 2.010 |
Table2.
Characterizations for the sputtered AlN films.
Table options
-->

Download as CSV
Sputtering condition | Deposition rate (nm/s) | Actual thickness (nm) | FWHM values of XRC (arcsec) | RMS (nm) | |
(0002)-plane | (10$ {bar {1}} $2)-plane | ||||
2000 W, 100 sccm, 550 °C | 0.210 | 213.77 | 360 | 3229 | 0.852 |
2000 W, 100 sccm, 600 °C | 0.216 | 219.72 | 270 | 2941 | 1.510 |
2000 W, 100 sccm, 650 °C | 0.233 | 237.42 | 191 | 2714 | 2.200 |
2000 W, 100 sccm, 700 °C | 0.197 | 200.70 | 130 | 2491 | 0.988 |
3000 W, 100 sccm, 550 °C | 0.330 | 207.93 | 184 | 3301 | 0.850 |
3000 W, 100 sccm, 600 °C | 0.340 | 214.19 | 241 | 2923 | 0.995 |
3000 W, 100 sccm, 650 °C | 0.346 | 218.17 | 205 | 2664 | 1.060 |
3000 W, 100 sccm, 700 °C | 0.316 | 199.31 | 119 | 2584 | 0.955 |
2000 W, 150 sccm, 600 °C | 0.206 | 209.28 | 371 | 2776 | 1.860 |
2000 W, 180 sccm, 600 °C | 0.218 | 220.40 | 349 | 2743 | 1.930 |
3000 W, 150 sccm, 600 °C | 0.315 | 198.23 | 292 | 2966 | 1.970 |
3000 W, 180 sccm, 600 °C | 0.322 | 202.65 | 410 | 3049 | 2.010 |
3.
Results and discussion
3.1
The effect of substrate temperature
Fig. 1 shows the (0002)- and (10




class="figure_img" id="Figure1"/>
Download
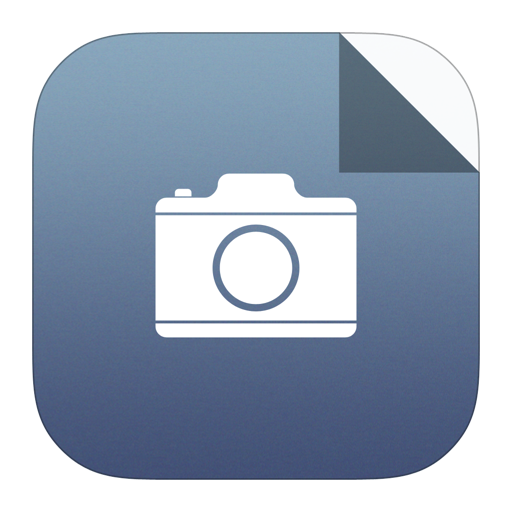
Larger image
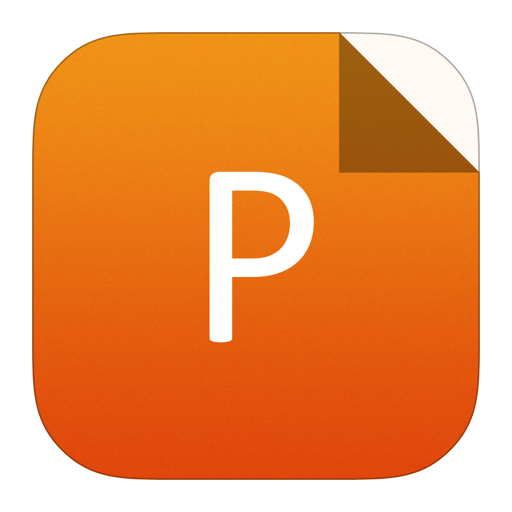
PowerPoint slide
Figure1.
(Color online) The (a) (0002)- and (b) (10
To better comprehend the impact of substrate temperature on the crystallinity of annealed AlN films, AlN films with various substrate temperatures are applied. Fig. 2 shows the effect of substrate temperature on the FWHM values of (10





class="figure_img" id="Figure2"/>
Download
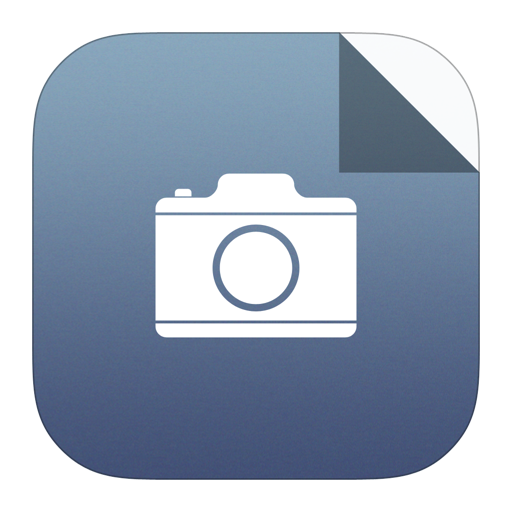
Larger image
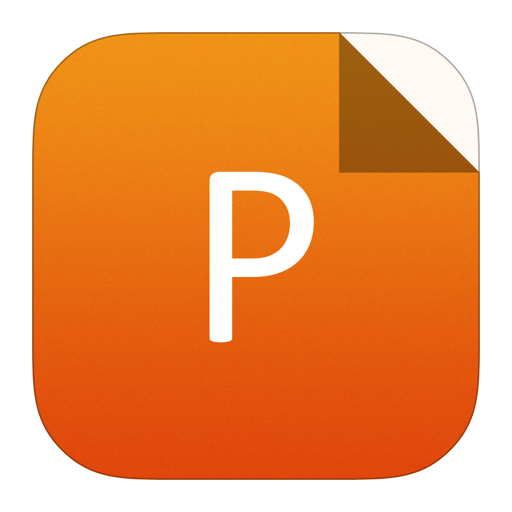
PowerPoint slide
Figure2.
(Color online) The FWHM values of (10
The relationship between the FWHM values of (10




class="figure_img" id="Figure3"/>
Download
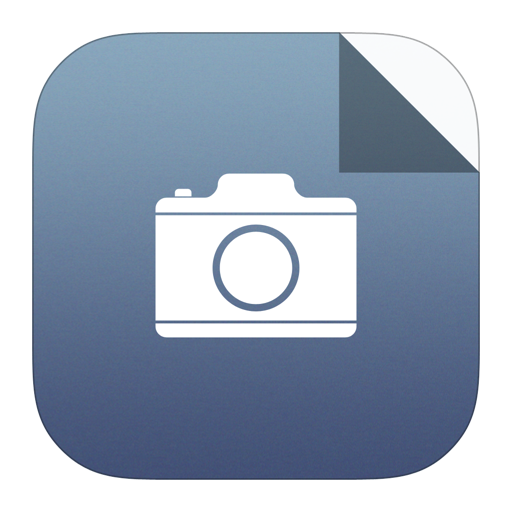
Larger image
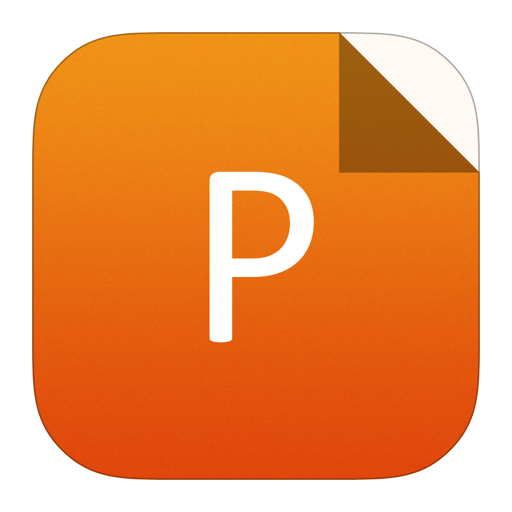
PowerPoint slide
Figure3.
(Color online) The (0002)-plane XRCs of the AlN films sputtered at (a) 2000 and (b) 3000 W with various substrate temperatures before HT annealing.
Figs. 4(a) and 4(b) show the Raman spectrum of AlN films sputtered at various temperatures before annealing. The E2(high) peak frequency is presented in Fig. 4(c). The peak intensity of strain-free AlN films was reported at the phonon frequency of 657.4 cm–1[24]. The smaller (larger) peak frequency indicates a larger tensile (compressive) stress. Before annealing, the E2(high) peak frequency of AlN films sputtered at 2000 W is gradually increased from 654.686 to 660.488 cm–1 as the substrate temperature increases. This means that the stress state of sputtered AlN films can be modulated from tensile to compressive stress by increasing the substrate temperature[25]. It is possibly because of the less vacancy density and dislocation density with higher sputter temperature. The 3000 W case has the similar tendency. However, the stress becomes from tensile to compressive at 600 °C because of less vacancy density and less dislocation density than the 2000 W case. After HT annealing, all the E2(high) peak frequencies of AlN films are higher than 657.4 cm–1 and similar, implying all AlN films have compressive stress after annealing due to the lattice and thermal expansion mismatch between sapphire and AlN in the recrystallization process[26].

class="figure_img" id="Figure4"/>
Download
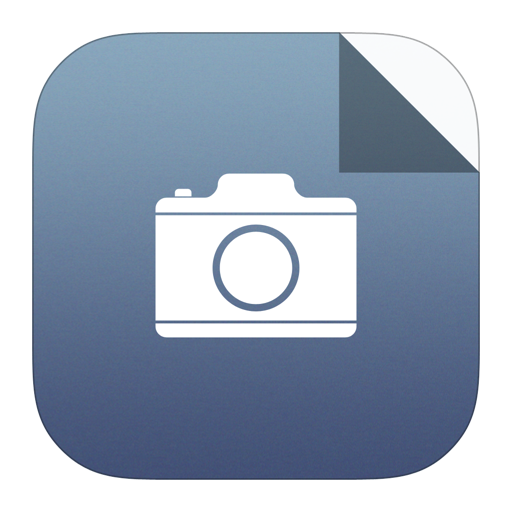
Larger image
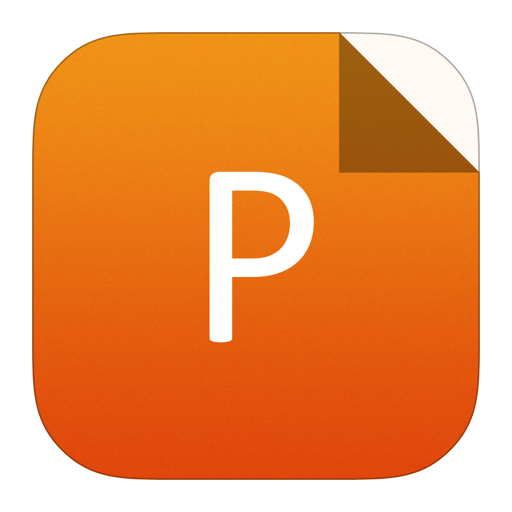
PowerPoint slide
Figure4.
(Color online) Raman spectrum of the AlN films sputtered at (a) 2000 and (b) 3000 W, a N2 flux of 100 sccm, and various substrate temperatures before annealing. (c) The E2(high) peak frequency of sputtered and annealed AlN films with different substrate temperatures.
Fig. 5 shows AFM images (5 × 5 μm2) of the AlN films sputtered at 2000 W and a N2 flux of 100 sccm with different substrate temperatures before and after HT annealing. The surface morphologies of the sputtered AlN films show high-density and uniform column structures in Fig. 5(a)[17]. The diameter and altitude of the columns are increased as the substrate temperature increases from 550 to 650 °C (increased size and number of white dots on the surfaces in these images), which is also demonstrated by the enlarged root mean square (RMS) from 0.852 to 2.20 nm. This may happen because the high substrate temperature will promote the deposition rate and increase the grain size of the AlN column[23]. However, as the substrate temperature further increases to 700 °C, the surface morphology is improved with small-size columns and RMS is reduced to 0.988 nm possibly because the reduced deposition rate and the increased nucleation density at the very start of sputtering. The 3000 W case has a similar trend (not shown here). After HT annealing, the column structures coalesce and a few pits (defects marked in the images) are observed, which were also mentioned in previous work[17]. For the annealed AlN films with low substrate temperature in Figs. 5(e) and 5(f), step-bunching morphology is formed after annealing, and sparse high-altitude columns still exist on the surface and decreases with increasing substrate temperature. When the substrate temperature increases to 700 °C, step morphologies with the RMS of 0.275 nm appear free of high-altitude columns in Fig. 6(h). All of the surfaces of samples with 3000 W after HT annealing have step morphologies (not shown here). The significant improvement of surface morphology after HT annealing is attributed to the coalescence of columnar domains and the annihilation of domain boundaries[17].

class="figure_img" id="Figure5"/>
Download
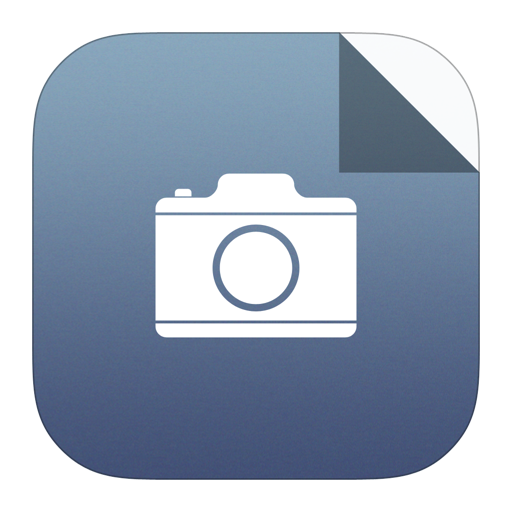
Larger image
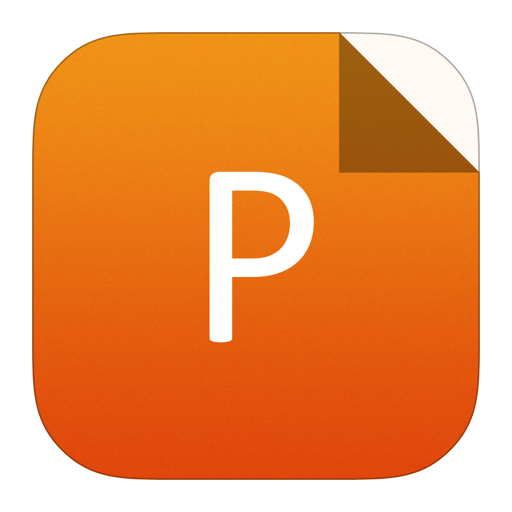
PowerPoint slide
Figure5.
(Color online) 5 × 5 μm2 AFM images of the AlN films sputtered at 2000 W, a N2 flux of 100 sccm and substrate temperatures of (a) 550, (b) 600, (c) 650 and (d) 700 °C before annealing and (e) 550, (f) 600, (g) 650 and (h) 700 °C after annealing.

class="figure_img" id="Figure6"/>
Download
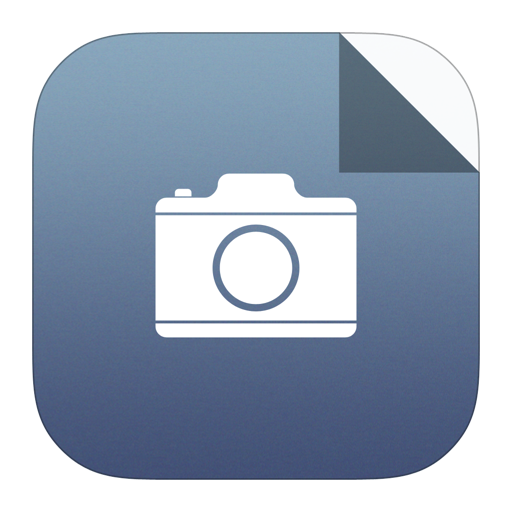
Larger image
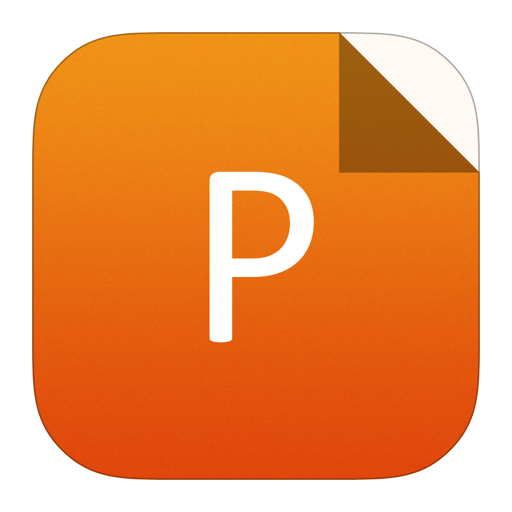
PowerPoint slide
Figure6.
The effect of N2 flux on the FWHM values of (10
3.2
The effect of N2 flux
N2 has two functions during the sputtering process, involving that (1) N2 is ionized as N source to bombard the Al target and form AlN and (2) N2 acts as environment gas and collides with sputtered particles. To reveal the effect of N2 flux during the sputtering process on the crystallinity of annealed AlN films, three different N2 fluxes of 100, 150, 180 sccm are used to deposit AlN at 600 °C. Fig. 6 shows the FWHM values of (10



To further analyze the crystallinity evolution of annealed AlN films with the increase of N2 flux during sputtering, the tilt component of sputtered AlN films is investigated. Fig. 7 shows the (0002)-plane XRCs of the AlN films sputtered with various N2 fluxes at 600 °C, 2000 W and 3000 W before annealing. For the AlN films sputtered at 2000 W, all samples have side peaks. Furthermore, the normalized intensity of side peaks decrease with decreasing N2 flux, which means the less tilt component. The 3000 W case has a similar tendency. During sputtering, the process pressure is decreased as the decrease of N2 flux. This will result in a longer mean free path of particles, which means that sputtered Al particles from Al target have less possibility to collide with N2 molecules from target to substrate. Thus, less energy of the sputtered Al will be lost in this process and AlN will deposit on the surface with higher energy, which will lead to less tilt component. This lower tilt component will possibly improve the vacancy diffusion. Hence, the FWHM values of (10


class="figure_img" id="Figure7"/>
Download
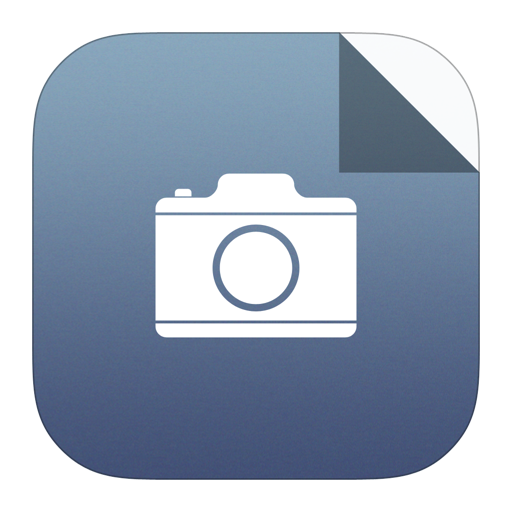
Larger image
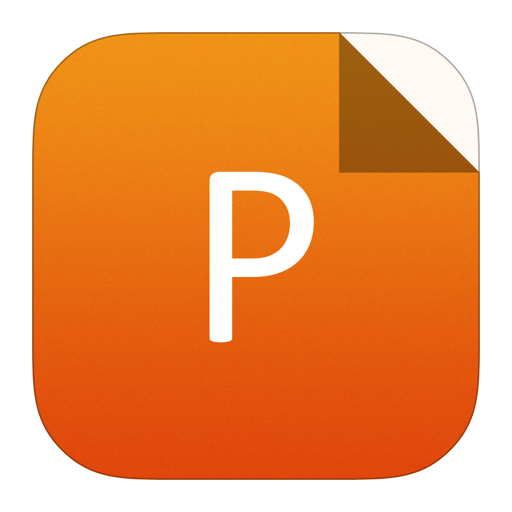
PowerPoint slide
Figure7.
(Color online) The (0002)-plane XRCs of the AlN films sputtered at (a) 2000 and (b) 3000 W before annealing with various N2 fluxes.
Fig. 8 presents AFM images (5 × 5 μm2) of the sputtered AlN films sputtered at 2000 W, 600 °C with various N2 fluxes before and after HT annealing. Before annealing, the size and altitude of column structures are increased as the increase of N2 flux and the RMS values are increased from 1.51 to 1.93 nm. After HT annealing, step-bunching structures are formed due to the coalescence of column structures. Moreover, high-altitude columns tend to disappear with the increase of N2 flux. However, lots of voids are observed for the annealed AlN films with a N2 flux of 180 sccm, which are ascribed to the remained residual spaces after the coalescence of domains[17].

class="figure_img" id="Figure8"/>
Download
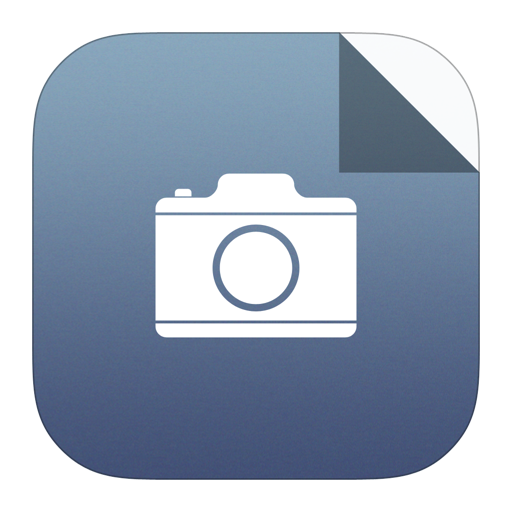
Larger image
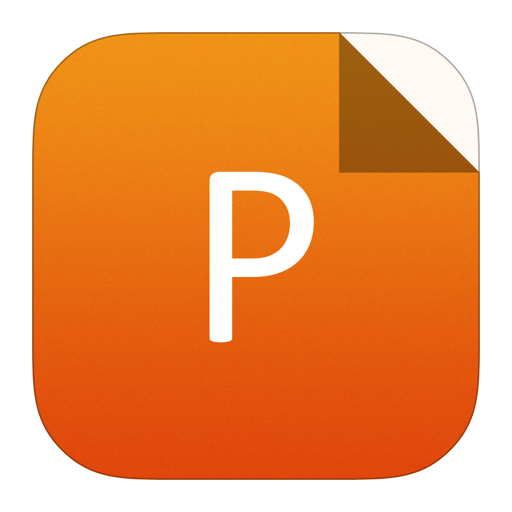
PowerPoint slide
Figure8.
(Color online) AFM images of the AlN films sputtered at 2000 W and 600 °C with N2 fluxes of (a) 100, (b) 150 and (c) 180 sccm before annealing and (d) 100, (e) 150 and (f) 180 sccm after annealing.
3.3
The effect of RF power
The effect of RF power can be analyzed by comparing the 2000 and 3000 W cases in the previous parts. Fig. 3 and Fig. 7 show that the 3000 W case has narrower (0002)-plane XRC of sputtered AlN films than 2000 W case with the same other sputtering conditions. It is because the higher RF power provides high kinetic energy for AlN during sputtering which lead to less tilt component. However, the FWHM values of (10

By further reducing the RF power to 1000 W, the color of sputtered AlN films will change from transparent to light yellow due to the lower deposition energy (not shown here). The FWHM values of (10

4.
Conclusion
The effect of sputtering parameters on the annealed AlN films is comprehensively studied. The high-quality annealed AlN films are obtained at lower substrate temperature, lower N2 flux, and lower RF power. The lower substrate temperature and lower RF power provide less energy for AlN deposition during sputtering, which leads to the increased tilt component and high vacancy concentration. A high vacancy will increase dislocation annihilation by improving dislocation climb during HT annealing. However, a vacancy concentration that is too high or too much of a tilt component will prevent the vacancy from diffusion or stop the dislocation climb. The transformation from compressive stress to tensile stress of sputtered AlN films may also be useful for AlN columns to twist. Lower N2 flux reduces the energy loss by decreasing the collision possibility of sputtered Al particles and N2 molecules, and thus improves the tilt component, which will improve vacancy diffusion. Finally, the FWHM of the (0002) and (10

Acknowledgements
This work was supported by the National Key R&D Program of China (Nos. 2016YFB0400800 and 2017YFB0404202), the National Natural Sciences Foundation of China (Grant Nos. 61527814, 61674147, 61904176, U1505253), Beijing Nova Program Z181100006218007 and Youth Innovation Promotion Association CAS 2017157.