Plate tectonics has come to explain the dynamics of nearly everything we observe at the surface: the kinematics of how plates move relative to each other, the correlation of ancient biogeographic provinces now in distant lands, and the drift of continents through the climatic belts. We know that such plate motions are achieved by subduction and seafloor spreading that are related to mantle downwelling and upwelling, respectively. We also know that plate tectonics is in fact the modern mode of mantle convection. But even as we know that the mantle is somehow involved in plate tectonics, how exactly it is, still remains largely a mystery. Evidence for surface plate motions affecting Earth’s deep interior remain in short supply.
According to new research, the mystery of plate tectonics in the deep Earth is beginning to be revealed. Published in the leading journalNature Geoscience, the research examined the chemical and isotopic compositions of rocks sourced from thousands of kilometers below the surface to better understand how Earth’s mantle responds to plate movements that occur near its surface.
In recent decades, seismology has revealed that the deepest mantle, right above the core-mantle boundary, is anything but homogenous. In fact, there are two vast structures sitting on the core-mantle boundary opposite to each other, one under the Pacific plate and one under the African plate (McNamara and Zhong, 2005). Some scientists have even called them “blobs”. These two blobs are characterized by slow seismic velocities and are termed large low shear-wave velocity provinces (LLSVPs). Although the precise origins of the LLSVPs remain debated, there is consensus that the blobs are chemically different from the ambient mantle that surrounds them.
How LLSVPs are related, or not, to plate tectonics remains unclear. Some researchers believe that the LLVPs are stable, ancient, and quasi-static structures that have maintained their same positions as the continents drift overhead over time (Dziewonski et al., 2010; Torsvik et al., 2010). Other researchers believe that the LLSVPs are dynamic structures that are intimately related to the subduction history of surface plate motions that influence, shape, and shift the deep mantle (Doucet et al., 2019; Mitchell et al., 2012). Finding ways to test between these hypotheses has proven difficult.
In the new study, leader author, Dr. Luc-Serge Doucet, and his international team of researchers, might have found a new way to interrogate the history of the deepest mantle. Even though much about LLSVPs remains mysterious, one thing has become clear: these thermochemical blobs tend to create mantle plumes at their edges. From Hawaii to Iceland, mantle plumes over the past 300 million years of plate reconstructions have been consistently demonstrated to originate from the edges of one of the two LLVPs (Evans, 2010; Torsvik et al., 2010), earning the edges of LLSVPs the name “plume generation zones”. The Hawaii plume rose from the Pacific LLSVP and the Iceland plume rose from the African LLSVP (Fig. 1).
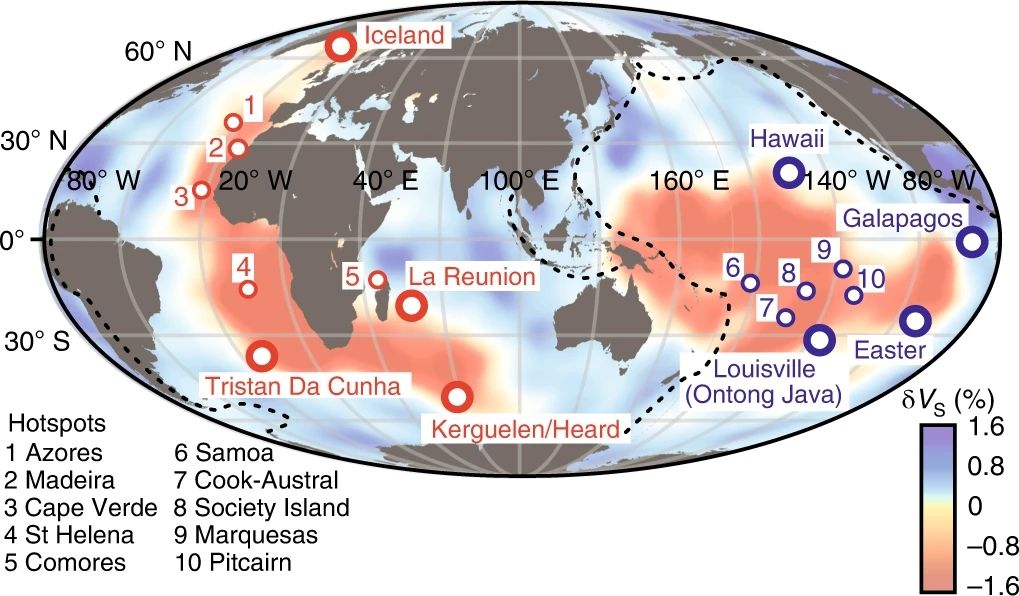
图1 源自两个地幔大低速省(LLSVP,图中红色阴影区域对应S波低速体)的地幔柱在地表形成一系列热点(Doucet et al., 2020)。研究表明,来自太平洋(蓝圈)和非洲(红圈)大低速省地幔柱产物的成分明显不同(Some mantle plumes forming hotspots at the surface originate deep in the mantle at the edge of one of the two LLSVPs (red shaded regions corresponding to slow shear-wave velocities). It turns out that the compositions of deep plumes derived from the Pacific (blue circles) and African (red circles) LLSVPs are distinct from each other.)
To make sure the plumes they investigated were indeed chemically linked to the LLSVPs at the base of the mantle, Doucet’s team had to be careful and selective. Not all mantle plumes originate from the deep mantle. The team used established criteria (Courtillot et al., 2003) to distinguish between shallow sources of mantle melt and those sourced from the deepest mantle that would most likely have a direct connection with the deep LLSVPs. Having selected a handful of deep mantle plumes for each of the two LLSVPs, they compared their geochemistry.
“Our team used trace metals such as lead,strontium,and neodymium, from hotspot volcanic islandsincluding theGalápagos Islands in the Pacific Oceanand Réunion Island in the Indian Ocean, to examine whether these two domains have the same chemical ‘make-up’,” Dr. Doucet said.
Prior to this most recent study, the two LLSVPs had been regarded as chemically identical. But what Doucet’s team discovered was just the opposite: the deep mantle is double-dealing, and the two LLSVPs are chemically distinct. “We found that the African domain was enriched by subducted continental materials, which was linked to the assembly and breakup of the supercontinent Pangaea, whereas no such feature was found in the Pacific domain,” Dr. Doucet said.
The chemical difference between the two mantle domains comes down to a difference in the influence of plate tectonics. After all, the two LLSVPs are separated by the Pacific “ring of fire”, a girdle of subduction along which most earthquakes and volcanoes occur on Earth. Professor Zheng-Xiang Li, co-author on the study, said the two chemically distinct hemispheres discovered by the team can best be explained by the distinct evolutionary histories of the two mantle domains during the Rodinia to Pangaea supercontinent cycles.
“We found that the African mantle domain contains continental materials, which were brought down by the subduction system for at least the past 600 million years. However, the Pacific mantle domain has been protected from the infiltration of such materials,” Professor Li said.
Due to the location and direction of subduction over the past hundreds of millions of years, the African mantle domain has been contaminated by subduction, but the Pacific domain has remained sheltered from it. During the assembly of supercontinent Pangaea, slabs subducted down over where the supercontinent would eventually reside. Then, after the supercontinent formed, subduction jumped to its edge, again diving beneath the position of the supercontinent. Thus, as deep mantle plumes sourced from the LLSVPs rise through the mantle, they encounter vastly different chemical reservoirs.
The new observation made by Doucet and colleagues is referred to as the deep mantle geochemical dichotomy (DMGD).
The research was co-authored by researchers from Curtin University in Australia, Tanta University in Egypt, St. Francis Xavier University in Canada, Université Libre de Bruxelles in Belgium, Queen’s University in Canada, and the Institute of Geology and Geophysics Chinese Academy of Sciences in Beijing.
Part of this summary comes from the Curtin University press release: https://news.curtin.edu.au/media-releases/new-curtin-research-uncovers-the-two-faces-of-the-earth/
主要参考文献
Courtillot V, Davaille A, Besse J, et al. Three distinct types of hotspots in the Earth’s mantle[J]. Earth and Planetary Science Letters, 2003, 205(3-4): 295-308. (Link)
Doucet L S, Li Z X, El Dien H G, et al. Distinct formation history for deep-mantle domains reflected in geochemical differences[J]. Nature Geoscience, 2020, 13(7): 511-515. (Link)
Doucet L S, Li Z X, Ernst R E, et al. Coupled supercontinent–mantle plume events evidenced by oceanic plume record[J]. Geology, 2020, 48(2): 159-163. (Link)
Dziewonski A M, Lekic V, Romanowicz B A. Mantle anchor structure: an argument for bottom up tectonics[J]. Earth and Planetary Science Letters, 2010, 299(1-2): 69-79. (Link)
Evans D A D. Proposal with a ring of diamonds[J]. Nature, 2010, 466(7304): 326-327. (Link)
McNamara A K, Zhong S. Thermochemical structures beneath Africa and the Pacific Ocean[J]. Nature, 2005, 437(7062): 1136-1139. (Link)
Mitchell R N, Kilian T M, Evans D A D. Supercontinent cycles and the calculation of absolute palaeolongitude in deep time[J]. Nature, 2012, 482(7384): 208-211. (Link)
Torsvik T H, Burke K, Steinberger B, et al. Diamonds sampled by plumes from the core–mantle boundary[J]. Nature, 2010, 466(7304): 352-355. (Link)
(撰稿:罗斯·米歇尔/岩石圈室;编辑:彭澎/岩石圈室)